The Discovery Channel
Why do Harvard doctors remain undaunted by the demands of discovery?
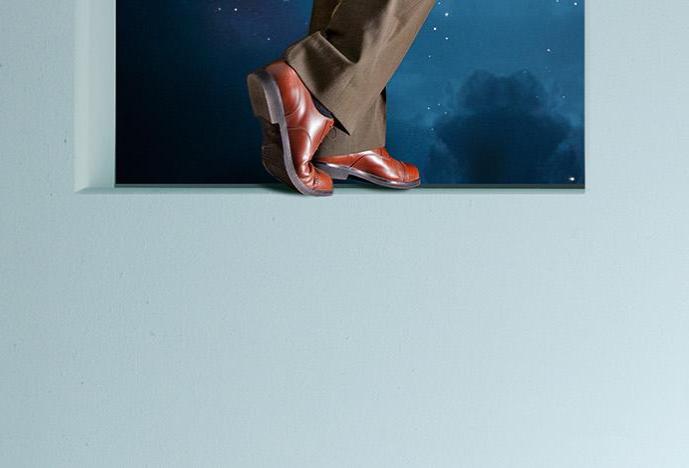
On one ridge stand scientists clutching discoveries rich with possibility; along another are physicians reaching for therapies to alleviate their patients’ suffering. Between them runs an abyss, its floor strewn with abandoned drug candidates and failed clinical trials.
Into this chasm ventured two unsuspecting Harvard Medical School neurobiology professors, Clifford Woolf and Bruce Bean. After years of collaboration, the scientists wanted to use their experience in deciphering the neurophysiology of pain to develop a new generation of analgesics. Joining forces with a group of neurobiologists and clinicians, they sought venture capital. In 2006, the group launched Solace Pharmaceuticals with a plan to translate several key discoveries into new drugs—and to bridge the gaps between bench and bedside, basic and applied science, academia and the pharmaceutical industry.
Neither Woolf nor Bean had attempted anything like this before, and they found that the journey from idea to drug carried a steeper learning curve and more steps—seeking a patent, licensing the technology, raising money for further testing, among many others—than either had anticipated. Nevertheless, their plan seemed to proceed flawlessly, and they quickly identified a compound that relieved pain in rats. Then came the giant hurdle of translation to human clinical trials.
“The results were beautifully clear,” says Woolf. “The drug didn’t work in humans.”
The researchers had experienced a common obstacle: molecular processes in animals don’t necessarily translate to human biology. Their drug failed in a proof-of-principle trial and never made it to more extensive phase II and III trials. Even if the drug had shown promise, other obstacles—including safety, regulatory, and financial ones—might well have derailed their work down the road. For every new drug that makes it to market, at least a hundred promising compounds fail at an experimental stage. Many biomedical researchers put the chances of a discovery surviving the trip to market more simply: it is a journey through the Valley of Death.
And academics are ill equipped to traverse that valley alone. “Medical schools aren’t set up to do small-scale proof-of-principle human clinical trials, much less large-scale ones, and scientists aren’t used to navigating regulatory issues,” says Jeffrey Flier, dean of HMS. “Those capacities lie in industry.”
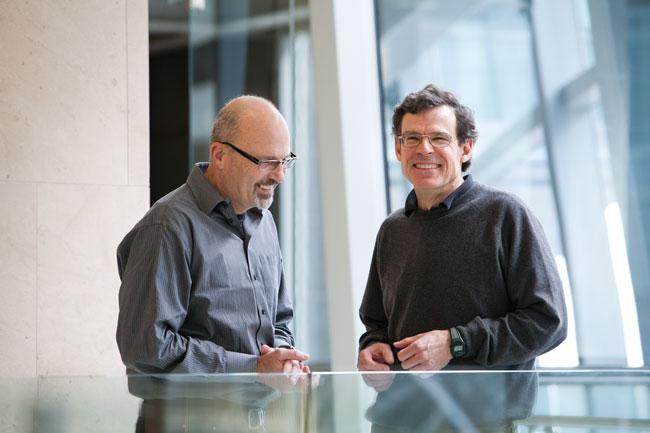
But the road can be treacherous for the pharmaceutical industry as well. Nine of ten potential medicines entering clinical trials fail. High-profile, late-stage drug failures have cost Big Pharma billions of dollars and prompted the industry to seek new, innovative approaches to drug discovery and development. These approaches include closer collaborations with academic institutions, from which, says Flier, “most of the fundamental discoveries come.”
Meanwhile, many brilliant university-based discoveries with true drug-development potential “just get left on the pages of medical journals,” says Frances Jensen, an HMS professor of neurology and director of epilepsy research at Children’s Hospital Boston. “At the basic science level, scientists might do great research, get their results published in a high-profile journal, and then move on to the next fundamental experiment. Their discovery just sits on a shelf. Nobody picks it up, and nobody takes the next step of translation.”
Even when promising ideas get noticed, universities lack the resources and know-how to develop them. The continued shrinking of research dollars adds more pressure to an already competitive process. And industry is cutting budgets as well.
These forces have created what one HMS official calls “a perfect storm.” But the School is creating shelters for those willing to brave the tempest.
At the Center of Discovery
Around the time that Solace halted clinical trials of the pain medication that Woolf and Bean had developed, the National Institutes of Health was unveiling an initiative—eventually expected to surpass $500 million a year—to address the very issues that had caused the researchers grief. Universities and medical centers around the country began to vie for a share of the funds, with the hope of using them to create clinical and translational science centers, or CTSCs, designed to ease the journey from discovery to therapy.
In 2007, Flier had barely settled in his new position as dean of HMS when he learned that the NIH would be phasing out the general clinical research center grants historically awarded to Harvard’s major teaching hospitals. Instead, HMS would have to apply for funding to create a CTSC to be shared with its hospital affiliates and the rest of the University. Flier pulled together a team that, under the leadership of Lee Nadler ’73, crafted a winning proposal for a five-year, $117.7-million award for such a center, now known as Harvard Catalyst.
The difference between translational medicine and basic research is intent, Flier says. In basic research, scientists seek to understand fundamental biology: how a certain part of a cell works, for example, or how a molecular signal passes from cell to cell. In translational work, researchers want to frame basic science findings to capture a disease’s cause or to improve its diagnosis, treatment, management, or prevention.
“We’re still a long way from even a basic grasp of the mechanisms that underlie most human diseases,” Flier says. “Even when we have that understanding, as we do with cystic fibrosis, for example, it’s not an easy leap to therapies. Turning discoveries into effective treatments is daunting, even before you encounter issues in funding, conflict of interest, regulation, or a host of other arenas.”
To help bridge that gap, in the past few years Flier created two new posts—dean for clinical and translational research, held by Nadler, and executive dean for research, held by William Chin ’72, both of whom brought significant experience in translational research and human clinical experimentation.
“Medical schools have at their heart a desire to help patients,” says Chin. “We focus on basic research—using such model systems as mice, zebrafish, and fruit flies—because we need this information for enough insight to learn what leads to human disease in the first place. But remember, one of our primary goals is to alleviate human suffering caused by disease, and stopping at basic research would leave us short of that goal. So while we don’t require scientists to take a translational approach, we encourage them to think about it. We’re also working to make the process easier for those who do.”
Sometimes, adds Nadler, the hardest step is the first one. “My colleagues who are basic scientists often tell me, ‘I’d really like to pursue translational research. But I don’t know what the question should be or how to proceed to the answer.’”
So Nadler, under the auspices of Harvard Catalyst, has fostered initiatives aimed at helping to frame those questions. One such initiative was a recent crowdsourcing challengethat solicited ideas—and funded research projects—based on a simple question: What do we not know to cure type 1 diabetes? This question was posed to the entire community of basic researchers, disease- and patient-focused clinicians, and technology innovators, and the wisdom of the crowd provided new approaches to an old and still vexing disease. “The effort was highly successful,” says Nadler, “in that it allowed people who had never worked in the field of type 1 diabetes to share their ideas.”
Chin agrees that collaboration is key to the School’s translational research initiatives. “We want to foster more productive multidisciplinary, interdepartmental, and cross-institutional work and team formation,” he says. “We believe this will lead to more innovation and creativity.”
But that goal bumps up against another hurdle to translational research—the culture of science. “When basic scientists have a translatable discovery,” Jensen says, “they generally have to hand that discovery over to a translation scientist and then to a clinician.” She adds that the incentives in academic medicine—journal publications, grants, awards, promotions—often reward individuals rather than teams. In contrast, translational and clinical work require more collaboration. “It’s hard,” she says, “for translational researchers to show their added value and get credit for their work.”
Part of the solution, Nadler says, is to evolve the culture and incentives. “What we need is a precise question, a team of investigators with the diverse skills to solve the problem, the required tools and technologies, and incentives to bring people from disparate disciplines together. If we say we need an answer to a specific problem and then provide pilot funding for that research,” he says, “we give collaborations more focus and means.”
As part of its incentive-building program, Harvard Catalyst provides one-year, $50,000 pilot grants for investigations that carry a high risk of failure yet offer big payoffs if successful. “But there’s a catch,” says Nadler. “You can’t work with anyone you’ve worked with before and you must reach across the University system to find a collaborative partner.” In three funding cycles thus far, the program has awarded 161 grants for innovative projects aimed at, for example, engineering resistance to epileptic seizures, identifying biomarkers for Alzheimer’s disease, and pinpointing neural indicators of dyslexia in infants.
Harvard Catalyst has also introduced practical courses in clinical research and translational medicine, including a weeklong introduction to clinical investigation, offered several times a year, and more advanced classes. Several affiliated hospitals offer training as well.
“Through such initiatives,” says Chin, “we’re creating a new breed of investigator. Basic scientists are engaged in learning about disease, and physicians are gaining backgrounds in basic science.”
Another program that provides critical assistance to foster and advance translational research at HMS is the Technology Development Accelerator Fund, launched and operated by Harvard’s Office of Technology Development. Fueled by donations raised from philanthropic sources, the Accelerator Fund is a grant-based program that enables investigators to bridge the often insurmountable development gap in order to establish proof-of-principle and to transform promising early-stage inventions into viable candidates for development, commercialization, and clinical application. The Accelerator Fund has provided seed funding for 27 projects at the level of approximately $200,000 each.
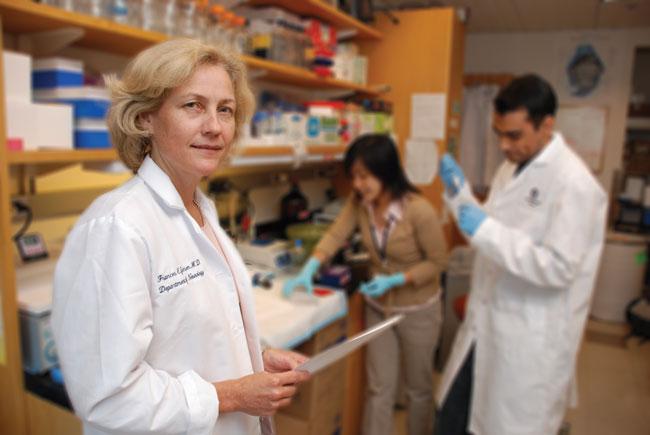
“Our primary motivation is to accelerate the development of promising new inventions that might otherwise languish for lack of support and validation, and to ensure that they’re expeditiously translated for the public good,” says Isaac Kohlberg, the University’s chief technology development officer. “We can’t predict or pick ‘winners’ with certainty. It’s all about planting the right seeds, nurturing the seedlings, encouraging them to blossom, and weeding when necessary. Every now and then we’ll be rewarded with a prize-winning flower, but we need to seed and cultivate constantly.”
Tending the Gap
Jensen had no such assistance when she ventured into the foreign landscape of translational medicine two decades ago, on a search for a new drug to control seizures in newborns. She had long been fascinated by synaptic plasticity—how synapses, or the connections between neurons, grow with experience. Now she wanted to apply her knowledge to heal injuries to the infant brain.
Such an approach—translating findings on rodent brains into aids for human infants—requires a delicate pairing of basic researcher and clinician. “But in my department, as in many departments, the clinicians don’t know many of the basic scientists all that well,” Jensen says. The gulf between the laboratory and clinic, researcher and physician, and animal model and human patient is a major obstacle in the translational journey. Yet increasingly, people on both sides of the gap are working to bridge it.
“As clinical medicine advances and as diagnostic tools, such as biomarkers and genetics, continue their rapid evolution, we’ll discover new areas of science, ones that you have to go to the bench to study,” Jensen says. “In some cases we’re discovering that some pathways involved in one disease process are surprisingly also important in another, seemingly unrelated disease process”—or, as Jensen’s work on controlling seizures in infants shows, that a drug intended for one disease may be effective against a vastly different disease.
Medications that control seizures in adults, for example, work only half the time in infants. From research in animal models, Jensen knew that antiseizure drugs work by targeting inhibitory synapses. But infant brains have fewer inhibitory and more excitatory synapses than adult brains do. “If you give babies a drug that targets inhibitory receptors,” Jensen says, “you’ll find they don’t have enough receptors for the drug to work.”
A number of laboratories had shown that lowering the number of chloride receptors inside infant brain cells causes them to respond to a drug that targets inhibitory synapses. It turned out that bumetanide, a long-used, federally approved diuretic, targets chloride receptors in the kidney. These receptors also are found in human brains, with the numbers found in infant brains surpassing those in adult brains. To win the U.S. Food and Drug Administration’s approval for repurposing this drug for infant epilepsy, Jensen and her colleagues had to prove that the drug targeted neural chloride receptors in animal brains, and then show that such receptors were also in the human brain.
Jensen’s team then encountered the first of several regulatory hurdles that precede clinical trials—the institutional review board, or IRB, which ensures patient safety. Because Jensen’s trial would involve three Harvard hospitals— Brigham and Women’s, Children’s, and Massachusetts General—it needed approvals from three separate IRBs. “It took about 18 months,” Jensen recalls. That process has since been streamlined through a combined IRB, thanks to Harvard Catalyst.
Jensen hopes to tap this eased process when a new mechanism she is investigating, one that blocks the progression to epilepsy, moves from animal to human studies. But since this mechanism might be a new rather than repurposed agent, yet more steps—involving, for instance, medicinal chemistry, toxicology, and drug metabolism—may be required. For those steps, academic institutions, which rarely have the needed expertise or infrastructure, often need to contract with industry.
Captains of Industry
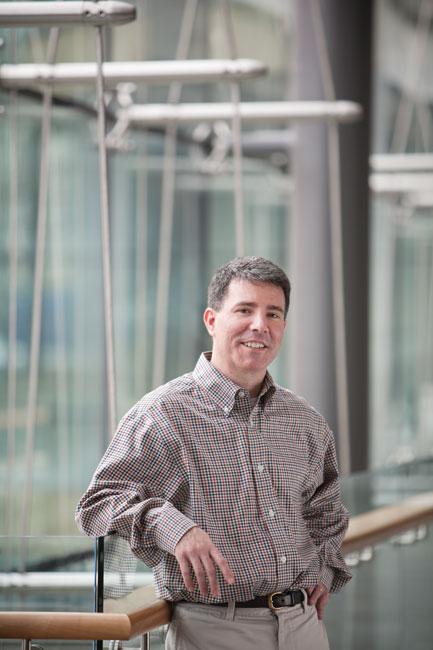
Randall King ’97 and Daniel Finley stumbled upon those limitations while shepherding a new molecule, one that helps rid human brain cells of the misfolded proteins implicated in Alzheimer’s and other neurodegenerative diseases, through the gauntlet needed to prove its investment value to potential industry collaborators.
King and Finley were exploring why ubiquitin, a small regulatory protein that normally helps neurons dispose of misfolded proteins, sometimes fails. Wayward proteins accumulate, forming the signature plaques of neurodegenerative diseases. Finley, an HMS professor of cell biology, and King, an HMS associate professor of cell biology, discovered that, in cell cultures, the enzyme Usp14 slows the degradation of an Alzheimer’s disease–linked protein. So the researchers began to hunt for a Usp14 inhibitor.
They worked with Byung-Hoon Lee, an HMS research fellow in cell biology who, using the School’s Institute of Chemistry and Cell Biology–Longwood screening facility, developed a high-throughput–screening assay to search for molecules that inhibited only Usp14. The strongest candidate, christened IU1, easily entered both mouse and human cells in cultures and boosted ubiquitin’s beneficial activity. Although the results were good, they weren’t attractive enough for investors. “Pharmaceutical companies tend to have a weak appetite for risk,” says King.
To make their invention more viable for development and commercialization, the researchers needed to demonstrate that the molecule could penetrate neurons. And they needed to boost the molecule’s potency. Those steps required more medicinal chemistry than they could provide. It was a perfect project for the Accelerator Fund. Kohlberg, who oversees the program, says this early stage of technology development is where many promising university technologies run aground. “The Accelerator Fund enables early innovations to achieve preliminary validation,” he says, “and selects early-stage technologies that manifest significant translational promise.”
King and Finley now have a compound that not only can cross the blood-brain barrier but is also ten times more potent than the previous compound. “That work made the innovation more attractive to potential industry partners,” says King. As a result, OTD identified potential partners, held discussions with a number of industry groups, and recently concluded a major agreement with a biotech company, Proteostasis Therapeutics, for the development and commercialization of this technology.
“This progress would have been impossible without the Accelerator Fund,” Kohlberg says. “The fund not only supported the project, enabling the key medicinal chemistry work to be done, but also devised an effective intellectual property strategy and undertook a dedicated marketing and licensing program. All of these steps culminated in our now having a strong industry partner that’s committed to the commercial development of this technology and that can, we hope, take it to the clinic in the near future.” If all goes well, Finley points out, it will still be two to three years before the scientists can test their molecule in human studies.
Doctoring Peppers
Even after their disappointing first attempt, Clifford Woolf and Bruce Bean wanted to see their work result in pain medications. As they pondered new approaches, they came up with chili peppers.
Bean knew that capsaicin, the molecule that puts the sizzle in certain peppers, opens a channel called TRPV1 in pain-sensing nerve cells. A scientist seeking to alleviate pain in a research model would normally try to block this channel. But Bean and Woolf realized they could also use capsaicin as a way to guide and deliver a painkiller inside pain-sensing neurons without affecting muscles or other neurons. When considering which pain medication to pair with capsaicin for delivery, the researchers hit upon QX-314, a derivative of the common anesthetic lidocaine. Their vision: a capsaicin/QX-314 package delivered through local injection to ease the pain of childbirth and postsurgical pain.
The time seemed ripe to pursue an industry partner for development and commercialization. This time, OTD identified and held discussions with a number of established companies with relevant expertise. Those led to the negotiation and conclusion of a major licensing and collaboration agreement with Endo Pharmaceuticals, which will navigate the regulatory hurdles when it comes time to apply for safety trials in humans, possibly next year.
The imperatives of translational medicine and the importance of collaborating with industry are inextricably related, Kohlberg says. “With rare exception,” he adds, “it’s only through licensing and collaboration with industry partners that early-stage inventions made in academic labs can reach fruition and culminate in a new FDA-approved drug. And such a culmination in therapy represents in many ways the ultimate fulfillment of our core mission to serve the public interest.”
Another important feature of agreements with industry relates to intellectual property rights and the right to publish. “When industry funds research in our laboratories, we expect to be able to publish those findings,” says Flier. Industry has traditionally sought to sequester results as proprietary as often and as long as possible. More and more, though, industry realizes it’s important to publish results, and researchers acknowledge the need for patent protection.
“The right to disseminate research results is sacrosanct,” Kohlberg says. “In all of our agreements with industry, we make certain that the right to publish is inviolable—it is absolutely protected and guaranteed.”
Previously, industry and academia each believed it could do everything alone. “In the past, partnering with industry was a bit frowned upon,” Jensen recalls. “But actually, it’s a necessary relationship. How else can drugs get to patients? Medical schools aren’t set up to make drugs.”
As for Woolf and Bean’s first failed trek, not all was lost. In the reverse of a spinout, Woolf brought one of Solace’s programs back into his laboratory for further development. The path across the formerly formidable Valley of Death might just turn out to be a two-way street.
Conflicts of interest
It wasn’t precisely his bout with mononucleosis that led David Knipe into a career in virology. By the time Knipe, now the Higgins Professor of Microbiology and Molecular Genetics at HMS, lost a semester of college to the illness, he had been primed to become a virologist by a string of inspiring courses and a stint in a leading-edge laboratory. But the combination of illness and inspiration sealed that deal, involving Knipe in academia-based innovation, and now, with the aid of Harvard’s Office of Technology Development, a licensing agreement with a manufacturer that may produce a vaccine for herpes simplex 2, the cause of genital herpes.
In his HMS laboratory, Knipe developed a replication-deficient form of the virus. In mice, the mutant virus triggered an immune response without reproducing. Knipe’s hope is to develop a vaccine for humans based on the mutant. That task—and the clinical trials to test his innovation’s safety and efficacy—will fall to Sanofi Pasteur. “This vaccine needs to be tested in people,” says Knipe. “Without this agreement, that wouldn’t happen.”
At HMS, such academic–industry partnerships are carefully tended to guard against conflicts of interest, whether real or perceived. The School’s policy aims to ensure that academic research remains robust and unfettered, and that industry involvement remains collaborative and fruitful. Overall, the goal is to translate ideas into powerful new medicines, devices, and technologies, a goal underscored by Jeffrey Flier, dean of Harvard Medical School, when he announced a revamped conflicts-of-interest policy last summer.
“Neither academia nor industry alone is equipped to develop the therapies so desperately needed to eradicate diseases worldwide,” says Flier. “For this reason, we are committed to encouraging collaborations between our faculty and industry while ensuring the transparency of those relationships through a policy that further codifies and enforces our high standards.”
The revised policy upholds the ability of HMS faculty to license technology to pharmaceutical and biotechnology companies, hold equity in such companies, serve on their scientific boards, and establish new ventures. At the same time, the policy prohibits academic-industry links that might adversely influence the integrity of such ties. Faculty can no longer accept, for example, personal gifts or any travel and meals from industry, other than those received during allowed activities. As of July 2011, faculty members are barred from participating in industry speakers’ bureaus or accepting any speaking engagement that would dictate the faculty member’s presentation content.
In the realm of medical education, the policy reinforces restrictions barring sales and marketing representatives of medical drug, device, or supply companies from accessing medical students. Continuing medical education courses may no longer be sponsored by a single company, and industry advertising and exhibits at continuing medical education events are restricted.
The conflicts-of-interest policy addresses the challenges of academic-industry collaborations, says Flier, while maintaining the School’s commitment to advancing research in human diseases. To review the policy, visit the Integrity in Academic Medicine website.
Cathryn DeLude is a freelance science writer.
Images: Anne Cutting (top); John Soares (Woolf & Bean, R. King); Patrick Bibbins (F. Jensen)