The Good that Viruses Do
The surprising and beneficial contributions that viruses bring to life
- 15 minute read
- Feature
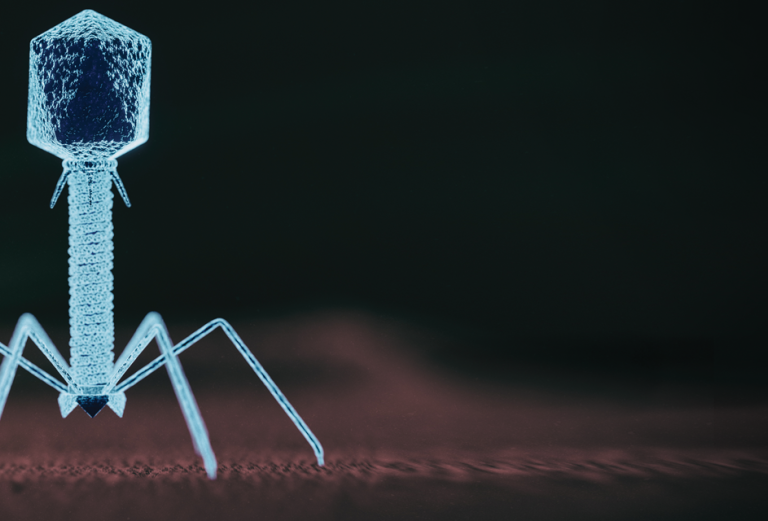
Paris, 1917. Hospitalized soldiers were dying from dysentery as Shigella bacteria overwhelmed their guts. Nothing could be done for them. Antibiotics wouldn’t be discovered for another decade.
Experimenting with Shigella cultured from the ill, microbiologist Félix d’Hérelle uncovered a difference between samples from patients who survived and those who succumbed. In survivors, an entity too small to be seen through his microscope was killing the bacteria. He called the attackers bacteriophages, or bacteria eaters.
D’Hérelle recognized that the mysterious phages offered a way to fight bacterial infections. In 1919, he isolated phages from Salmonella bacteria that were causing a typhoid outbreak in chickens and used them to cure the birds. A few months later he thought he would risk treating a boy with a dire case of dysentery. First, however, d’Hérelle and his team drank a concoction of phages they’d isolated from another dysentery patient. When no one felt the worse for wear, they gave it to the boy.
He recovered.
Advances in microscopy later revealed what phages really are: viruses that infect bacteria and single-celled microbes known as archaea while ignoring plants and animals.
Endeavors like d’Hérelle’s have helped show humanity that viruses can provide medical and research benefits.
#NotAllViruses
Although some two hundred kinds of viruses are known to infect, sicken, or kill us, as the emergence of SARS-CoV-2 has most recently hammered home, that’s only one part of the picture. Viruses also keep us alive. They form part of the body’s microbiome and safeguard our health. They can be harnessed to treat illness, deliver vaccines, and diagnose infections. They’re wielded as research tools to illuminate biology and disease and develop new drugs. We can thank snippets of viral genomes, incorporated into our DNA tens of millions of years ago, for how our reproductive and nervous systems work.
We have these amazing approved therapies, yet we’re just scratching the surface of what viruses can do to modify and treat diseases.
Protovirus components likely even contributed to the emergence of life on Earth, and viruses continue to drive evolution today. They form a crucial part of the global ecosystem that allows us to survive.
“We can’t generalize viruses as being harmful,” says Mohammadsharif (Sharif) Tabebordbar, PhD ’16, who led the development of an experimental gene therapy that uses modified virus components.
“Despite the devastating effects of viral diseases, the viruses that count most in our lives are crucial not in disease but in health and in all aspects of life,” says Eugene Koonin, an expert on the genetics of evolution and viruses at the National Institutes of Health’s National Center for Biotechnology Information.
While scientists and physicians across the HMS community study ways to combat the viruses that plague us, other colleagues are uncovering and exploiting viruses’ potential for good. Some, like Connie Cepko, the Bullard Professor of Genetics and Neuroscience in the Blavatnik Institute at HMS, do both.
“We love viruses for all of our work,” says Cepko. Her lab members have devised virus-based tools to map circuits in the brain, prolong vision in mouse models of inherited blindness, and test for SARS-CoV-2.
“Viruses are useful in a ton of ways in research and the clinic,” agrees Timothy Lu, MD ’10, an associate professor of biological engineering and electrical engineering and computer science at MIT and CEO at Senti Biosciences. “We have these amazing, approved therapies, yet we’re just scratching the surface of what viruses can do to modify and treat diseases.”
The old is new again
Strangely, the idea of phages as treatments has never overcome initial skepticism. Outside of areas such as eastern Europe, the medical community discarded them when antibiotics emerged mid-century. Today, however, phage-based therapies are gaining traction.
That’s partly because phages kill bacteria in a different way from antibiotics, offering a potential lifeline as antibiotic resistance plays a role in the deaths of 5 million people each year worldwide. Phages also offer pinpoint targeting, since most phages evolved to infect one or a few strains of bacteria or archaea. Identifying the bacterium causing a patient’s illness and finding a phage that kills it could wipe out the troublemaker and leave beneficial bacteria unharmed.
In the past few years, doctors operating under compassionate use allowances have saved a small number of people from life-threatening bacterial infections that defied all other treatments. The number of phage therapy clinical trials is ticking upward. In 2021, the U.S. FDA and the National Institute of Allergy and Infectious Diseases awarded $2.5 million in grants to groups developing phage-based therapies.
As an MD-PhD student, Lu gravitated toward phage engineering after learning about the problem of antibiotic resistance. “I thought it was crazy we didn’t have a solution,” he recalls. In advisor James Collins’s lab at Boston University, Lu “got really intrigued by phages. They had this weird vibe of being an old technology but with this whole new toolset.”
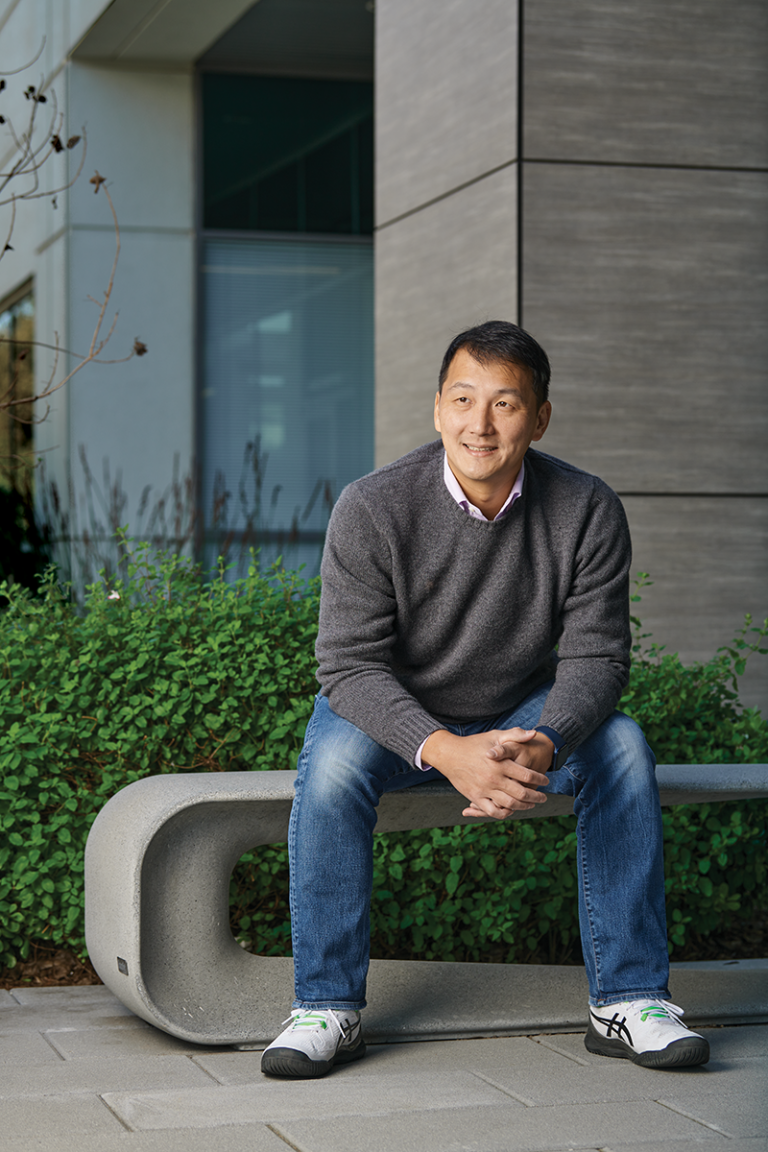
Collins and Lu showed that phages can break up biofilms, stubborn webs of bacteria and extracellular matrix that immune cells and antibiotics have difficulty penetrating. Modifying the phages to deliver genes into bacteria to enhance the activity of antibiotics and adding enzymes from other phages that “chew up” biofilm matrices produced even better results. Harking back to d’Hérelle, in 2019 Lu and colleagues at Massachusetts General Hospital built an intestine organoid and showed how a phage they’d isolated from Shigella vanquished infection.
Phages’ potential is vast, but finding the right ones isn’t easy. Each case requires combing through meager phage libraries or sampling places where the bacterium lives, such as in sewage. Often, the hunt fails or yields phages that are difficult to work with. Synthetic biologists like Lu would love to prompt one type of phage to open multiple bacterial doors, but there’s limited space inside phage “bodies” to cram genetic keys. Though these hurdles and more lie ahead, Lu and others in the field are optimistic that they’re surmountable.
Not in my human
Another group of researchers is interested in viruses that infect humans without causing disease and then fend off more dangerous viruses and bacteria. GB virus C, an asymptomatic blood-borne virus, slows progression to AIDS in people with HIV and lowers the risk that infection with Ebola virus will prove deadly. Mouse studies suggest that certain innocuous herpesviruses and cytomegaloviruses prevent infection by Listeria and Yersinia pestis, which causes bubonic plague. Even harmful viruses can harbor disease-combating strategies for scientists to adapt. The hepatitis A virus can protect against hepatitis C, and researchers have used lymphoma-associated viruses to cure type 1 diabetes in mice.
Proponents lament that human viruses remain underexplored as infection fighters. “Medicine may benefit from taking mutualistic viruses more seriously,” reads a 2011 article in Nature Reviews Microbiology titled “The good viruses.”
Subscribe to Harvard Medicine magazine
Oncology has had better luck. Records spanning millennia tell of unusual cases where infection with what we now know to be viruses that cause diseases such as influenza, chicken pox, and measles temporarily beat back people’s cancers. After a century of attempts with sometimes disastrous results, researchers in the past decade have succeeded in safely wielding viruses to cure or curb cancers or sensitize tumors to other therapies.
Oncolytic viruses—from onco, meaning mass or tumor, and lysis, to break apart—work by killing cancer cells and by helping the immune system do so. Unlike chemotherapy, radiation, and surgery, oncolytic viruses go after cancer cells while largely sparing healthy cells. Researchers defang the viruses so they don’t cause disease themselves and can engineer them to deliver molecules that draw immune cells to the tumors.
The only oncolytic virus approved in the United States, called T-VEC, earned FDA clearance in 2015. It’s a modified herpesvirus injected into late-stage melanomas in the skin and lymph nodes. Dozens of other candidates are being tested for treatment of numerous cancers. They may work solo, in tandem with conventional treatments, or when combined with advanced immunotherapies such as checkpoint inhibitors. The main challenge, researchers say, is stopping the immune system from destroying the viruses before they do their work.
“When I started my PhD, clinicians thought we were nuts for wanting to put viruses into people,” says Lu. “Now it’s a totally different world. People have seen that this type of engineering can transform patients’ lives.”
Your package has been delivered
Cepko went to college in the ’70s and fell in love—with viruses.
“I appreciated their wiliness, their different life cycles, their ways of escaping host surveillance,” she says. “I thought they were super interesting and valuable to study.”
All that viral variety serves one goal: to latch onto cells, inject them with DNA or RNA, and turn those cells into virus copy machines. As doctors and scientists sought new and better ways to get treatments into patients’ cells, particularly newfangled gene therapies, Cepko joined them in asking why not take advantage of viruses’ innate skills.
Now, after decades of trial and error, viruses have become indispensable medical delivery vehicles.
I appreciated their wiliness, their different life cycles, their ways of escaping host surveillance. I thought they were super interesting.
“Gene therapy would be way, way, way behind if we didn’t use viruses and viral vectors,” says Cepko.
Researchers first disarm the virus by removing some or all of its genome from inside the virus’s protein shell, or capsid. They might tweak capsid proteins to generate less of an unwanted immune attack or to home in on certain cell types. Then they fill the hollowed-out capsid with whatever they want to insert into cells, be it a healthy copy of a defective gene, genome editing machinery, a drug, or a vaccine.
The FDA issued its first gene therapy approval in 2017. Additional approvals have followed, and clinical trials have shot up into the thousands. So far all the gene therapies licensed worldwide, which have saved the lives of tens of thousands of adults and children with otherwise untreatable and often terminal diseases, use viral vectors in some way.
Hollowed-out adenoviruses and adeno-associated viruses, or AAVs, are the most popular choices for injecting healthy genes into the body. That’s how approved gene therapies for spinal muscular atrophy and a form of inherited vision loss known as retinal dystrophy work. Cepko’s lab has engineered AAVs to deliver gene cocktails into the eye to mop up inflammatory, oxidative, and metabolic damage caused by hereditary retinal degeneration. The therapy has successfully prolonged vision in mouse models. This spring, the lab partnered with a biotech company that secured the retinal dystrophy approval to take Cepko’s therapy forward.
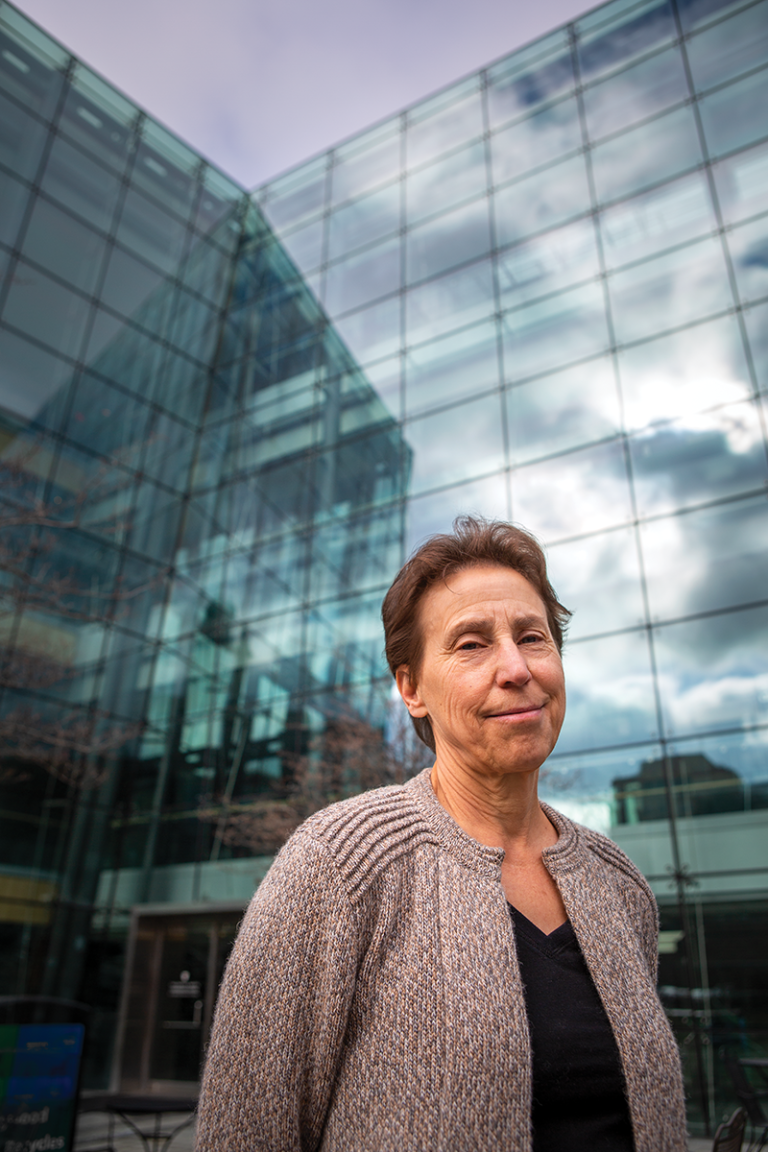
“We haven’t treated people yet, so I can’t really say, but I would be very proud if we could help anybody retain their vision for any amount of time,” she says.
When researchers want to change the genomes of cells permanently, such as to teach bone marrow stem cells to make nonsickled hemoglobin, they turn to modified lentiviruses and retroviruses: viruses that don’t just inject free-floating genetic material into the cell nuclei they infect but actually integrate their genes into a host cell’s DNA. These treatments take place outside the body. In the FDA-approved CAR-T cell therapies that revolutionized cancer treatment in recent years, clinicians retrieve T cells from the blood of patients with particular types of cancer and send the cells to a lab, where technicians use viral vectors to deliver a gene that helps the cells attack that cancer. The augmented T cells are then multiplied and reinfused into the patient. Similar strategies are being explored for blood disorders, HIV/AIDS, and dozens of other conditions.
Although promising, viral vector-based gene therapies still present challenges. Capsid proteins sometimes instigate immune response. As with phage capsids, AAV capsids have limited cargo space. Viral vectors introduced systemically accumulate in the liver, with only a small percentage reaching the tissues that need treatment. The low uptake means patients must receive massive doses to get enough vectors where they’re supposed to go, which can cause fatal liver toxicity. Finally, costs are astronomical—up to $2 million per treatment—raising concerns about equitable access.
Tabebordbar and collaborators may at least have solved the liver issue for the treatment of genetic muscle diseases.
Personal inspiration
Tabebordbar grew up watching his father slowly lose muscle strength and coordination from a rare genetic disease. The decline motivated Tabebordbar to become a scientist and pursue treatments for muscle-wasting conditions. His PhD advisor, Amy Wagers, the Forst Family Professor of Stem Cell and Regenerative Biology at Harvard and HMS, inspired him to seek solutions in gene therapy.
The breakthrough came when Tabebordbar was a postdoctoral fellow in the lab of Pardis Sabeti, MD ’06, a professor of organismic and evolutionary biology and of immunology and infectious diseases at Harvard and a member of the Broad Institute of MIT and Harvard. Tabebordbar and a pan-Harvard team including Sabeti and Wagers generated more than 5 million slightly differing AAV capsid variants and introduced them into animal models. They identified the 30,000 capsid types that reached muscle tissue, evolved those into new variations, and identified a family of capsids that zeroed in on muscle and mostly bypassed the liver. The vectors, dubbed MyoAAV, worked at one-hundredth the doses currently given to people. The team published the results in September 2021 in Cell.
The work gives researchers a protocol for developing liver-sparing AAV capsids that reach tissues other than muscle. Sabeti’s group has already attempted one for the central nervous system, which Cepko is testing in the retina. Tabebordbar, meanwhile, spun off a company to take MyoAAV to human trials and now serves as its chief scientific officer. Although his father’s disease has progressed too far for him to benefit, Tabebordbar hopes the therapy will prove safe and effective for thousands of others with genetic muscle disorders.
“It’s exciting to engineer these viruses to develop technologies that can help humanity,” he says.
Viral vectors also have become a hot topic for researchers developing vaccines. Half a dozen have been approved worldwide, all targeting SARS-CoV-2 or Ebola virus. Among them is Janssen Pharmaceuticals’ COVID-19 vaccine, the development of which drew upon years of work by Dan Barouch, MD ’99, the William Bosworth Castle Professor of Medicine at HMS and Beth Israel Deaconess Medical Center. Barouch had been working on an AAV-based vaccine for HIV, then redirected the project when the new pandemic struck.
It’s exciting to engineer these viruses to develop technologies that can help humanity.
Researchers are testing other viral vector-based vaccines for intractable pathogens, including Zika virus and malaria parasites. Bacteriophages may aid in this arena, too. A team including Richard Sidman, MD ’53, the HMS Bullard Professor of Neuropathology, Emeritus, published a proof-of-concept study in PNAS in 2021 showing the promise of using phages to deliver COVID-19 vaccines.
Long-term tenants
Astronomer Carl Sagan used to say, “We are made of star stuff.” Recent years have shown that we are also made of microbes. Some estimates hold that bacteria in and on our bodies outnumber our own cells 10 to 1. Now scientists say we may harbor a tenfold greater number of viruses.
The rise of metagenomic sequencing in the past fifteen years has allowed scientists to identify a good chunk of the viruses that comprise our virome, says NIH’s Koonin. Understanding what those viruses are doing should follow. Early studies suggest that while some cause damage or await opportunities to do so, others do us favors, including modulating our microbiomes and fighting off invaders. Such findings have led to speculation that manipulating the virome could help treat gastrointestinal and mood disorders.
Other viruses go deeper, down to our DNA. Every so often in the far recesses of history as humans and our evolutionary forebears evolved, a retrovirus infected an egg or sperm cell; that cell became a fertilized embryo that developed to term with viral DNA incorporated everywhere; and the resulting offspring went on to have offspring of their own, passing the DNA through subsequent generations. According to Koonin, about 50 percent of our genome once belonged to viruses and related mobile genetic elements.
Most of the viral gene remnants, or endogenous retroviruses, studied so far have proven either inert or latent, lurking until they reawaken and contribute to disease. An unknown number, however, were evolutionarily repurposed for our benefit. Mounting evidence suggests that endogenous retroviruses spurred the rise of placental mammals. Genes derived from viruses make proteins that form a foundational layer in the placenta and regulate a hormone that controls birth timing in primates. In the nervous system, endogenous retroviruses appear to contribute to brain development, long-term memory formation, and neuronal communication. Our once-viral genes also may influence immune function, embryonic development, and “probably a number of things we are not fully aware of yet,” says Koonin. More will surely resolve as researchers peer closer.
Evolution engines
The viruses that threaten humans and the animals and plants we’re familiar with barely register among the estimated 1023-1031 virus particles that dominate our planet, most of which stay busy infecting other microbes. Their effects on cellular life run deep.
Viruses cull microbes around the world in incredible numbers every day, creating sediment that sustains food chains, providing nutrients for photosynthetic marine organisms that produce half the world’s oxygen, and helping power carbon, nitrogen, and phosphorus cycles.
“Viruses basically regulate ecology and biogeochemistry on a global level,” says Koonin.
The so-called arms race, in which viruses spur hosts to develop antiviral defenses that then incite viruses to overcome those defenses and so on, represents one of the most powerful drivers of evolution on Earth, Koonin says, as do viruses’ abilities to transfer genes among one another and from host to host.
Learn more about the world of viruses
Although it seems intuitive that if viruses need cells to replicate, then they must have evolved after cells, observations and computational analyses have led a group of researchers including Koonin to the conclusion that certain building blocks of viruses predated the development of cellular life. Protoviral elements in the primordial soup formed RNA and DNA and ultimately laid the path for the evolution of cells, Koonin explains. Later, cells provided proteins for structures such as capsids; viral elements began to co-opt cells for replication; and modern viruses arrived.
“The emergence of complex life would not have been possible without contributions from genetic parasites in general and viruses in particular,” Koonin says.
Viruses basically regulate ecology and biogeochemistry on a global level.
From the origins of life to technologies that preserve it, learning about viruses can transform people’s opinions on what once seemed like straightforward agents of disease and death.
“When I hear the word virus, I’m not scared anymore,” says Tabebordbar. “I ask, ‘Okay, what type of virus? What does it do? What are the implications?’ ”
Even as their lives and careers warp around a pandemic virus, he and colleagues worldwide continue to unveil the good that viruses can do.
Stephanie Dutchen is manager of feature content and multimedia in the HMS Office of Communications and External Relations.
Images: Koto_Feja/iStock/Getty Images; Jay Watson (Lu); John Soares (Cepko)
Research at Harvard Medical School hangs in the balance due to the government’s decision to terminate large numbers of federally funded grants and contracts across Harvard University.