Internal Dialogues
If we take the time to listen, our bodies' cells have quite a bit to say
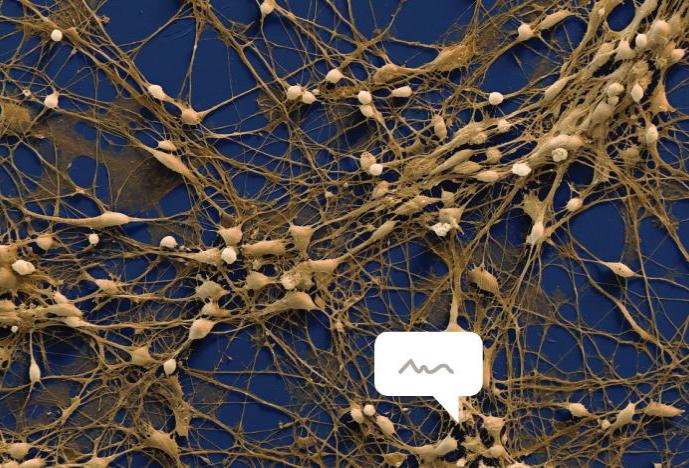
The chef shouts for more of the day’s specials. Immediately, certain line cooks start chopping onions while others heat oil on the stove. One cook nudges his neighbor for the salt. A sous-chef finishes plating salads and rings a bell. A waiter appears and ferries the plates into the dining room.
If the human body were a kitchen, its cells would be the chefs, wait staff, diners, and busboys that perform all the specialized tasks required to keep the organism running smoothly—only there would be 200 kinds of cells doing different jobs.
The key to successful functioning? Communication. If cells didn’t talk to one another, human bodies wouldn’t be able to see, hear, breathe, think, move, or do anything at all.
“Every aspect of an organism’s function depends on some kind of communication,” says Joan Brugge, the Louise Foote Pfeiffer Professor of Cell Biology at HMS.
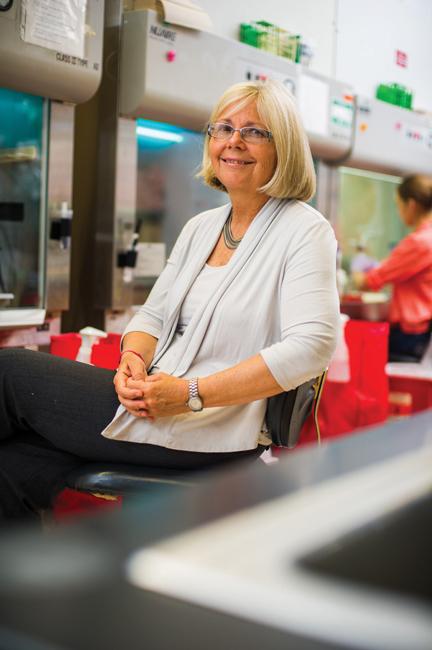
Whether it’s a group of insulin-producing cells calling for everyone to take up more sugar from the bloodstream, or a stem cell murmuring to its neighbors that they should become cartilage while those guys over there become muscle, intercellular communication tells every cell what to be, what to do, where to go, when to reproduce, and when to die.
It’s not surprising, then, that almost everything that goes wrong in our bodies can be traced to a failure of communication. Cells can go deaf, as in type 2 diabetes when they don’t respond to insulin. They can go mute, as in type 1 diabetes when pancreatic cells don’t release insulin at all. Cells can send the wrong message or misinterpret a correct one, speak up at inappropriate times, or act when they haven’t been told to.
Scientists have been trying to learn the ancient language of cells for more than a hundred years, eager to deepen the knowledge of how our bodies work.
Unfortunately, despite great progress, “We’re not yet fluent,” says John Flanagan, a professor of cell biology at HMS. “We can go to a restaurant, but we might order the wrong item. We can’t have a conversation.”
Flanagan anticipates a time when doctors and researchers can “listen in on what’s going wrong in a certain disease and then be able to direct change. We could say with confidence, ‘I want these cells to stop dividing.’ ”
Every time cell biologists think they’re close to understanding the syntax of the human body, the details seem to get more complicated. But with each passing year, Flanagan, Brugge, and others learn more about the conversations that keep our bodies running and devise new methods to eavesdrop on and interrupt diseases such as cancer. Their work takes us another step toward becoming conversant in our cells’ languages.
Are You Talking to Me?
Adrian Salic’s immune cells are communicating more than he’d like.
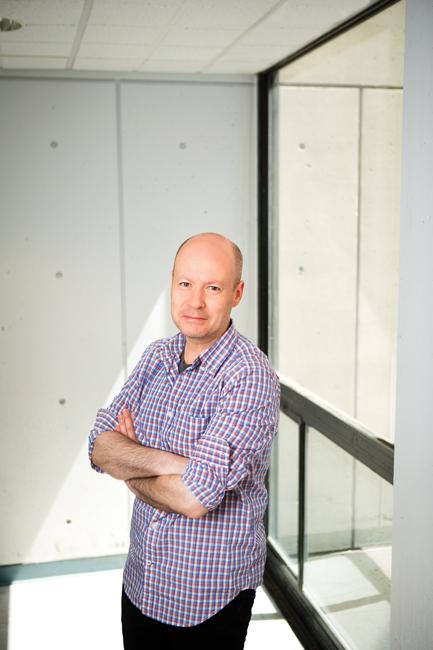
Pollen particles that Salic has inhaled have sounded an alarm. Mast cell-bound immunoglobulin E antibodies have begun to latch on to the particles and to call for reinforcements. Other proteins leap into the huddle until finally the mast cells burst, spilling out histamine and other inflammatory substances.
In other words, Salic, an HMS associate professor of cell biology, has seasonal allergies.
He rubs his eye. “One way to think of cell communication,” he says, “is as a set of languages with many dialects.”
Cell A talks by releasing a chemical signal in, say, Russian. The signal travels a predetermined distance and reaches Cell B, which can be next door or at the far end of the body.
Cell B hears the message because it has receptors on its surface that fit the signal’s highly specific molecular shape: It’s got an ear for Russian. It relays the signal through its membrane, interprets it, and responds.
Cells C and D don’t do anything because either they’re out of earshot, or they lack receptors to understand the language.
“I will not respond to Russian because I don’t speak Russian,” says Salic. “A cell that doesn’t have the receptor for a given signal won’t be able to interpret it.”
Compared with the world’s estimated 7,000 spoken languages, cells communicate using 20, including Notch, Jak-STAT, and Hippo. Each language has many dialects. The G protein-coupled receptor family, for example, includes a thousand different olfactory receptors, each dedicated to a specific smell.
Salic is learning to speak Hedgehog, a language crucial for stem cell differentiation and maintenance, which, if uttered too infrequently or too often, can cause birth defects or basal cell carcinoma. He builds tools that reveal in more detail how Hedgehog messages are created, sent, received, and translated into action.
Salic recently found that Hedgehog-speaking cells within the same tissue can be variably competent in the language. He’s now investigating what the biological significance of this finding might be.
Mother Tongue
Embryonic stem cells are born fluent in every language. As they differentiate, they lose receptors they don’t need, thus narrowing the number of languages they can articulate. A lung cell ends up with a different combination of receptors from that of a neuron.
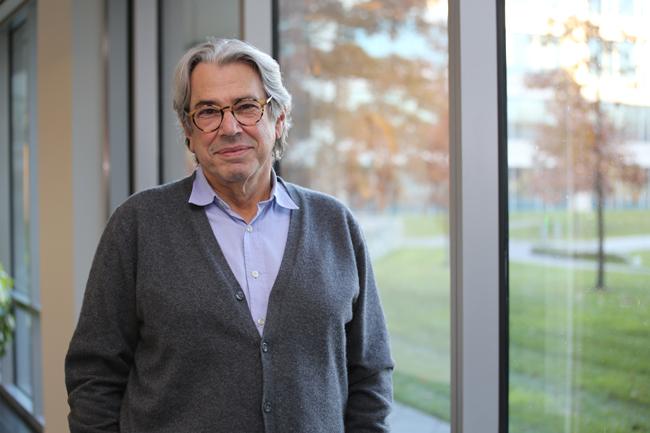
Spyros Artavanis-Tsakonas, an HMS professor emeritus of cell biology and the chief scientific officer at Biogen Idec in Cambridge, Massachusetts, has helped teach the world the grammar and syntax of one of those languages: Notch. He has identified many of the molecular components that allow cells to speak, hear, and act on Notch messages as they differentiate.
Lately, he’s dreamed of listening to all cell conversations, the equivalent of pinning microphones on every person on Earth.
“If you know that this protein talks to that one, which then talks to that one, and so on down a line of fifty proteins,” he says, “then if something goes wrong, you have not one drug target but fifty.”
Semaphore
Sometimes the language of cells is expressed not in words, but in signals. Many cells receive the same molecular signal but react in different ways. According to Flanagan, signals are more like spatial and temporal coordinates that cells use to make decisions.
“The brain and nervous system depend on a wiring diagram to function, like a computer. Except they have to wire themselves up,” says Flanagan. “It’s mind-boggling.”
Earlier in his career, Flanagan was one of the first to identify axon guidance cues. More recently, he found that they sometimes act like Goldilocks: at low concentrations, the cues will beckon developing axons closer, but at high concentrations, will warn them away. This push-pull positions the growing axons at just the right distance from the signal-emitting cells.
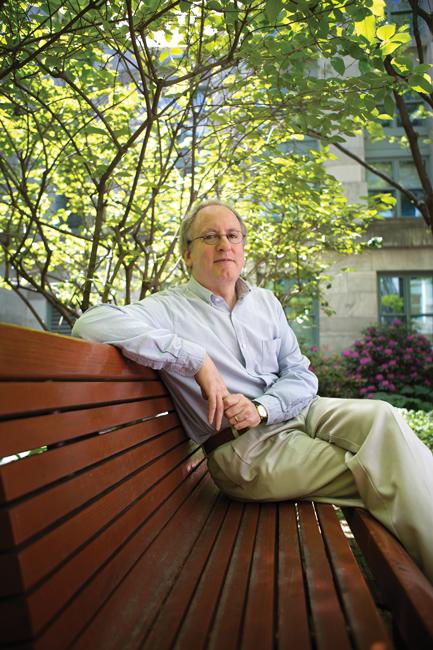
Flanagan hopes his research will uncover a clue for coaxing axons to regrow in adults after brain and spinal cord injuries. Scar tissue that forms after trauma contains cells that broadcast inhibitory signals to prevent the injury from spreading. Axons hear the “keep out” message and don’t regenerate in the injured tissue.
“We’re trying to develop a therapy that makes them ignore the signals,” says Flanagan.
Healthy cells listen to environmental cues. “The default for a cell is to die unless it receives the right signals from the outside,” says Brugge. Cancer cells, however, undergo a series of communication alterations that allows them to move into places they don’t belong and to proliferate when they should self-destruct.
Brugge recently discovered a sinister way environmental signals may shield breast cancer cells from chemotherapy. She developed a model that mimics the spherical structure of intraductal tumors surrounded by a matrix produced by normal cells. Treatment killed all the tumor cells except those in the outer layer.
Further studies revealed why: The surviving cancer cells touched the matrix, which whispered reassurances that they were in the right place.
“The signal from the matrix protects the cancer cells,” says Brugge. “The cancer cells sense they’re in their normal niche and rally a host of adaptations to withstand treatment.”
Like Flanagan, Brugge is now searching for a way to disrupt communications for the sake of healing.
Loose Talk
However cells speak and make decisions, they couldn’t function without a communications network.
Cells’ signals may be like radio signals, directed at particular cells or broadcast to an entire region. Receptors, therefore, are less like ears than like finely tuned receivers, clustered on an antenna called the primary cilium.
Ever since scientists started studying cell anatomy, “they’ve been fascinated by the beauty of the cilium,” says Salic. “It was an enigma. Now we know it impacts a huge number of developmental and physiological processes because so much signal transduction is concentrated there.”
Cell communication infrastructure has vulnerabilities that enemies can exploit. Doctors and researchers are learning how they, too, can hack or restore this network to thwart disease.
Brugge has found that a single ovarian tumor can contain many subpopulations of cancer cells. Crosstalk among these cells allows the tumor to metastasize, implant, and cause the buildup of abdominal fluid called ascites.
“We want to figure out what the critical communication nodes are so we can hit them and collapse the ecosystem,” says Brugge.
Some treatments replace a missing signal, like L-dopa does in people with Parkinson’s. Others, such as beta-blockers, disable the receiving mechanism.
“It’s like I try to call you and the number is out of service,” says Artavanis-Tsakonas.
Speak Up, Please
Dig deeper into cell communication and things quickly get complicated.
“Twenty-five years ago, we thought there was one signal for one receptor. Now we’re finding that each signal can have multiple receptors and that each receptor can receive multiple signals,” says Flanagan. “In the molecular era, it quickly became evident that signal transduction can be a bit of a nightmare.”
Once inside the cell, each message undergoes a children’s game of telephone, getting amplified, split, or otherwise chemically modified as it’s passed along one or more branched signaling pathways until it reaches the cell nucleus or an organelle. As if mapping those conversational flow charts wasn’t tricky enough, researchers must grapple with how cells listen simultaneously to multiple messages to make decisions.
“Signal integration is very important and very mysterious,” says Artavanis-Tsakonas.
These days, researchers painstakingly inactivate one gene at a time in a given cell to find out what cellular components are necessary for a signal to produce a response. Even when that response is “simply” to activate a gene, the product can change depending on RNA splicing, the action of noncoding RNAs, and other factors.
“In short, it’s complicated,” says Brugge. “We used to just look at mutations and DNA. Now we have to look at so many other variables to understand what leads to dramatically different cellular responses.”
But complexity has its advantages. Combining a limited group of signals in inventive ways allows organisms to develop a variety of biological processes—and to evolve.
That’s why Hedgehog, Notch, and other languages appear again and again in different contexts in the human body, says Salic.
And when all animals use the same cellular languages, a certain degree of flexibility explains “how the heck you can build a worm, a fly, and a frog” from them, says Artavanis-Tsakonas.
All this diversity of life from 20 languages. Combining them produces nature’s most beautiful literature.
Stephanie Dutchen is a science writer in the HMS Office of Communications and External Relations.
Images: Eye of Science/Science Source, Mattias Paludi (top); Sam Ogden/DFCI (J. Brugge); John Soares (A. Salic, J. Flanagan); Biogen Idec Media Services (S. Artavanis-Tsakonas)