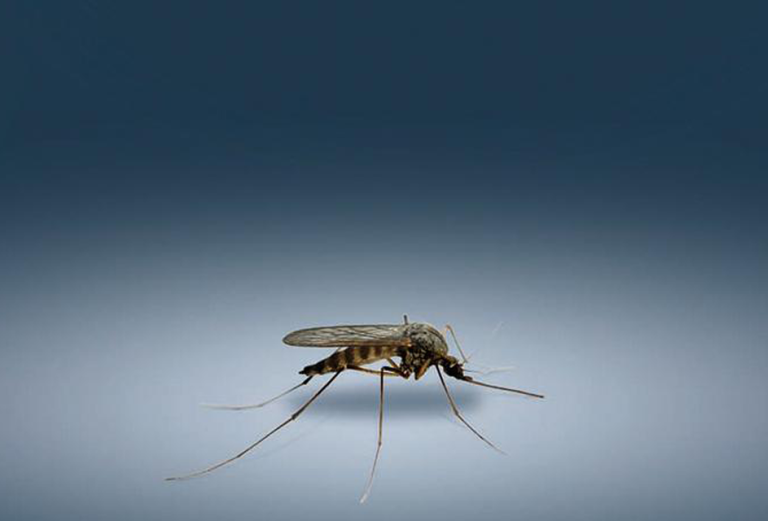
Invisible invaders have long lived among us. Bacteria, viruses, and parasites have had an upper hand as agents of sickness and death for much of our joint existence.
In sickrooms and on battlefields, antibiotics vanquished bacteria only in the past century. The victory seemed sweeping and miraculous. It was, however, short-lived: Bacteria are resilient pathogens that mutate to survive challenges to their well-being. Replicating every 20 minutes, these microbes have proven to be quick-change artists adept at thwarting drugs that target their weak points.
Viruses are equally mutable, although it is their genetic infidelity that makes them so slippery. A person infected with the human immunodeficiency virus (HIV) may carry multiple versions of the original virus, copies whose genetic makeups differ so markedly that no single drug can recognize them all.
Parasites, including the insidious Plasmodium falciparum, which causes malaria, evolved with humans in order to survive. We know this because they’ve left their fingerprints on our genome.
All told, antimicrobial drug resistance strains budgets and threatens some of the greatest successes in global health. In the United States, for example, treating antibiotic-resistant infections costs $20 billion per year.
Worldwide, up to one in five people diagnosed with HIV infection harbor a strain that is resistant to antiretroviral therapy, now the standard of care. Even antiviral drugs aimed at seasonal influenza are losing their punch, unable to topple influenza A.
Progress against malaria, which causes nearly one million deaths each year—90 percent of those among children in Africa—is hampered by emerging resistance to the only available antimalarial drug. The story is similar for tuberculosis: more than 50 countries have reported cases of extensively drug-resistant tuberculosis.
A new breed of researchers has emerged to counterattack the resistance efforts of these changeable microbes. Scientists are finding ways to reanimate so-called wonder drugs and to develop new technologies that reveal genetic vulnerabilities. These investigators peer back into evolutionary history and trace forward the microorganisms’ escape routes to discover ways to eradicate the pathogens and the infectious diseases they cause. But that’s not all. These investigators also seek to apply the lessons learned from a given pathogen to other microbial foes that evade our immune defenses.
In the Pink
For Bruce Walker, the challenge is HIV and the unusual patients called “elite controllers,” untreated patients who live with only trace levels of the virus that causes AIDS. He looks beyond the global pandemic.
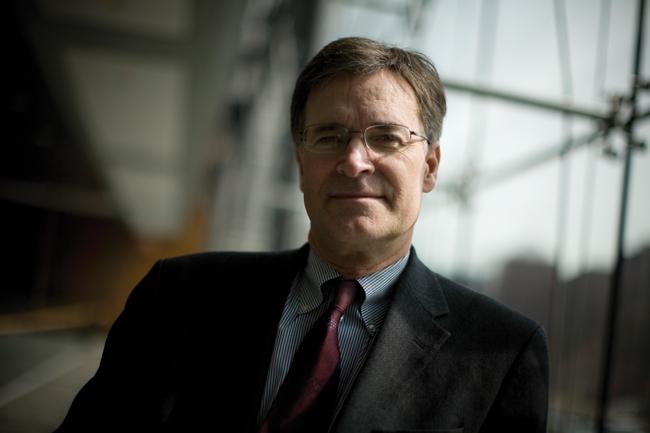
“I think HIV is giving us a window into the immune system,” says Walker, an HMS professor of medicine at Massachusetts General Hospital and director of the Ragon Institute. “If we’re talking about the future of medical interventions, I think the next decade is going to show incredible progress in terms of harnessing the immune system to prevent and cure human diseases.”
Research has produced drug cocktails that keep HIV infections in check. Scientists generally agree that combination therapies will likely be necessary to fend off other pathogens, too. As an example, Suzanne Walker, an HMS professor of microbiology and immunobiology, points to a once-successful group of antibiotics known as beta-lactams, which have been disarmed by a bacterial stratagem. Beta-lactams act by targeting the bacterial cell wall, disrupting how a cell divides, and disabling the way it grows and establishes an infection. To foil such attacks, bacteria that were once sensitive to beta-lactams changed; they became able to produce an enzyme that chews up the antibiotic before it reaches its target. How to reestablish beta-lactam’s effectiveness? One approach: create a drug that blocks the drug-eating enzyme. Once the enzyme is rendered ineffective, the first-line antibiotic works again. This, says Walker, is the strategy behind the composition of Augmentin, the pink liquid known to any parent with a child prone to ear infections. It combines beta-lactam with a defense against the aggressive enzyme.
Envelope, Please
Although rebooting beta-lactam by disabling the disruptive enzyme is effective against mild infections such as sinusitis, pneumonia, and bronchitis, it doesn’t faze tougher ones, such as MRSA.
Methicillin-resistant Staphylococcus aureus, named MRSA after the antibiotic it deflects, is one of the most virulent pathogens to plague hospitalized patients. Its facility in developing resistance has allowed it to evade all major classes of antibiotics. Suzanne Walker studies the cell envelope of S. aureus, the first line of defense for the organism, but also a point of vulnerability.
In their search for a way to target MRSA, Walker’s research team screened genes and proteins in S. aureus cells to understand steps along the resistance pathway—and to then find compounds that might work against another target. Together with scientists at the HMS Institute of Chemistry and Cell Biology–Longwood Screening Facility, Walker’s team singled out an effective chemical compound. A pharmaceutical company is now testing that compound along with other drug candidates that hit the same target.
“If you combine any one of these compounds—including ours—with beta-lactams, they overcome methicillin-resistant infections in animals,” Suzanne Walker says. “That’s pretty good.”
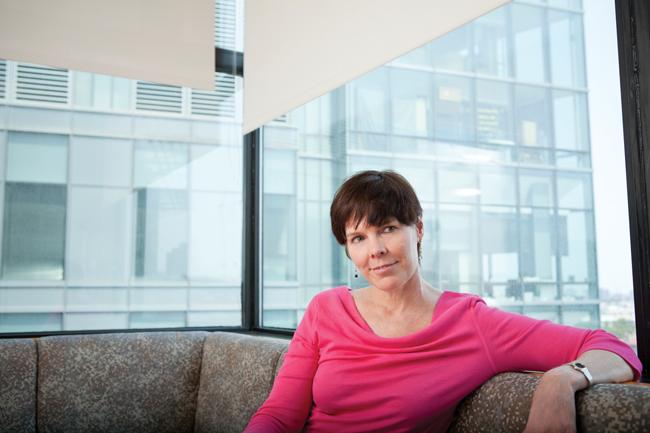
Walker is cautious when she talks about her lab’s discovery, pointing to the long history of short-lived successes in our battles against infectious diseases. A footnote to that history, in fact, proved pivotal to her decision to become a chemist. When Walker was nine years old, she heard it was possible to die from a hangnail that had become infected.
“I realized that little things used to kill people,” she says. “That was when I learned that antibiotics had not always existed.”
That revelation propelled Walker to study biological pathways and to pursue new ways to target infectious disease pathogens.
Particle Field
For Tim Lu ’10, the eye-opening career moment hit when he was a medical student on rotation at a VA hospital. He was struck by the number of chronic wound infections he was seeing and by the fact that patients returned again and again, never cured.
Lu, an assistant professor of biological engineering and electrical engineering at MIT, looked backward for innovation and found it in an area of biology and infectious disease study that had been shelved for nearly 80 years. He became fascinated by bacteriophages, viruses that were first identified in the early twentieth century. Capable of targeting bacteria in highly specific ways, phages have evolved in step with the bacteria they infect and consume. Yet they do not harm human cells.
More than a century ago, scientists sought to capitalize on this benevolence in studies that attempted to marshal the phages’ antimicrobial activity to halt bacterial infections in people. Sometimes the phages worked as the researchers hoped; other times they didn’t. This perceived unreliability caused bacteriophages to fall from favor as potential antimicrobials, a loss of interest hastened by the expanded use of the more broadly effective, and lifesaving, penicillin.
Those early hit-or-miss results, however, stemmed from a trait that now makes the phages relevant, Lu says. Bacteriophages naturally target certain species of bacteria, some narrowly and some broadly.
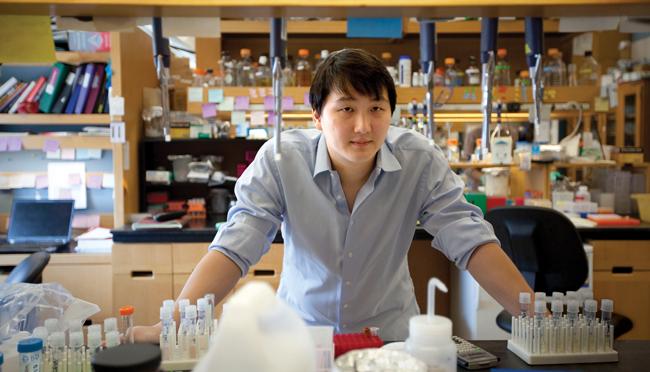
Bacteriophages have already proven their worth in molecular biology as tools for overproducing targeted genes or for picking out peptides, short chains of amino acids that have specific properties. Lu and others in the field see a wider potential for these small workhorses.
“Phages are a form of personalized therapy,” says Lu. “You can tune the bacteria that the phage likes to feed on.”
Tuning Fork
Bruce Walker also seeks to fine-tune systems, specifically the human immune system so that it will fight off HIV. He has witnessed how drug cocktails have changed the face of HIV infection since the early days of the epidemic, when he first saw AIDS patients while a resident at Mass General. After more than 30 years as a researcher in the field, Walker says, “HIV is gradually revealing its secrets.”
Among those secrets are the rare individuals who become infected but whose immune systems do not become overwhelmed by a high viral load. Some such people have been infected for more than 30 years and remain healthy without treatment. Scientists have learned that these elite controllers possess a genetic variation that keeps HIV at bay, holding it harmless in reservoirs within their bodies.
The same variation is also associated with a heightened risk for autoimmune diseases, kicking immune systems into high gear and making individuals vulnerable to disorders that cause the body to consider its own tissues to be foreign invaders, marked for destruction.
“The same thing that helps elite controllers potentially fight HIV makes one more susceptible to the cells of one’s own body,” Walker says. “To understand how it’s happening gives us the hope that we might be able to augment immune control of HIV in people who are not controlling it spontaneously.”
Drawing on analytic skills more typically applied to stock market movements, computational biologists working with Walker have discovered regions of striking rigidity in the virus—genetic material that has stayed stable in a sea of dynamic mutations. These stable regions might be good therapeutic targets. Other HMS researchers, in collaboration with scientists at Rockefeller University, have isolated potent HIV-neutralizing antibodies from elite controllers.
Knowing how the immune system functions in such powerful ways in some individuals may have broad implications for understanding how the immune system hides disease, Walker speculates.
“We don’t yet know how to induce those antibodies, but we do know that the body, under the right circumstances, can make them,” he says. “We just have to figure out how to initiate that response with a vaccine.”
Fitness Regimen
As with the genetic mutation that confers both HIV control and autoimmune disease risk, changes in genetic character also affect another infectious disease: malaria.
For more than a century, scientists have known that the mutation that causes sickle-cell disease, a red blood cell disorder, also protects people from malaria. This was the first elucidated example of natural selection in humans.
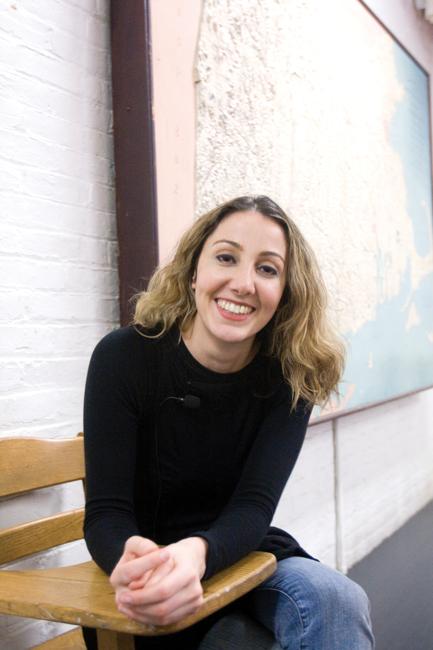
Pardis Sabeti ’06, an associate professor of organismic and evolutionary biology at Harvard University, studies genetic variation in the malaria parasite as it occurs through the forces of evolutionary pressure. Like other microbes, the malaria parasite has developed the ability to resist drugs. A program to eradicate the disease, launched in the 1950s, began to fail by the 1970s as the parasite developed resistance not only to chloroquine, the first-line treatment, but also to the insecticide DDT, an agent aimed at mosquitoes that carry the parasite. As each new mutation arose, the parasite evolved; newly mutated forms then spread worldwide. Sabeti calls this “evolution in action”—a demonstration of natural selection occurring in observable time.
“There’s a good percentage of the country that doesn’t believe in natural selection or evolution, but everybody understands the emergence of drug resistance,” Sabeti says. “These microbes are evolving, they’re changing, they’re developing resistance, and they’re spreading. And it’s happening all the time.”
Malaria is deeply challenging for another reason: the parasite needs humans as part of its life cycle. “Anytime you become a critical part of a microbe’s life cycle, it’s going to be a far bigger challenge to get it to give you up,” Sabeti adds.
Better knowledge of the malaria parasite’s changing genome can contribute to vaccine development and to better diagnostics. This knowledge can also be used to track which mutations lead to drug resistance to help inform which treatments to offer which patients.
“We have not exhausted our ability to get more information from understanding our defenses against malaria,” Sabeti says. “The more we understand how these microbes use their own diversity to their advantage, the better off we will be in our development of treatments, vaccines, and surveillance capacity.”
Suzanne Walker hedges when asked if she is hopeful about taming antibiotic resistance.
“I’m not unoptimistic, so long as people think differently about the problem. We need to take a variety of approaches and explore novel ideas,” she says.
The microbes—in all their diversity and novelty—won’t wait.
Elizabeth Cooney is a science writer in the HMS Office of Communications and External Relations.