The Twilight Zone
What discoveries were unimaginable even a decade ago?
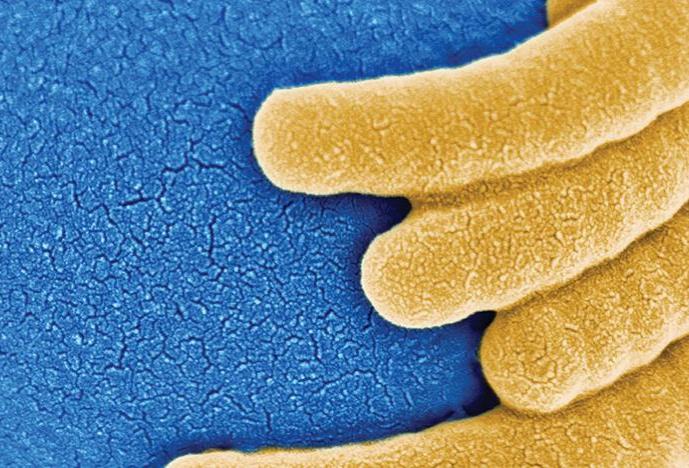
Templates from Nature
Realm of Imagination: Could DNA, that mighty molecule celebrated as the ultimate information source for biological beings, also be a font of data on nature’s form and function? It is to synthetic biologists. These investigators bring a Cubist’s eye to the natural world and, from each new vantage, discover unexpected therapeutic possibilities.
From Shadow to Substance: From this helical molecule, scientists such as William Shih, an HMS associate professor of biological chemistry and molecular pharmacology at the Wyss Institute for Biologically Inspired Engineering, tease out insights into design, stability, and self-assembly and use them to devise medical aids and treatments that are biologically effective, efficient, and sustainable. Shih and colleagues have, for example, designed scaffolds that help improve the control of enzyme-chaperoned reactions, constructed tubes and geodesic spheres that one day may ferry cancer drugs to tumor cells, and built nanoscale-sized components that, when injected into the body, could self-assemble as medical devices.
The Harvard Dimension:Advances in synthetic biology at the Wyss Institute happen, says Donald Ingber, director of the institute and the Judah Folkman Professor of Vascular Biology at HMS, because scientists think expansively, design creatively, and, with faculty and industry-seasoned technical experts, build cleverly to develop therapeutics in service to medicine. Work by Shih; Ingber; Peng Yin, an HMS assistant professor of systems biology; and other colleagues at the Wyss Institute has led to three-dimensional DNA that can be programmed to take almost any shape imaginable.
What’s Next? “We will move beyond DNA to the many new classes of RNA,” says Ingber, “and into gene and therapeutic delivery systems that will, for instance, be capable of changing the properties of cells and tissues in ways important to regenerating organs. Whatever we can imagine, we can build.”
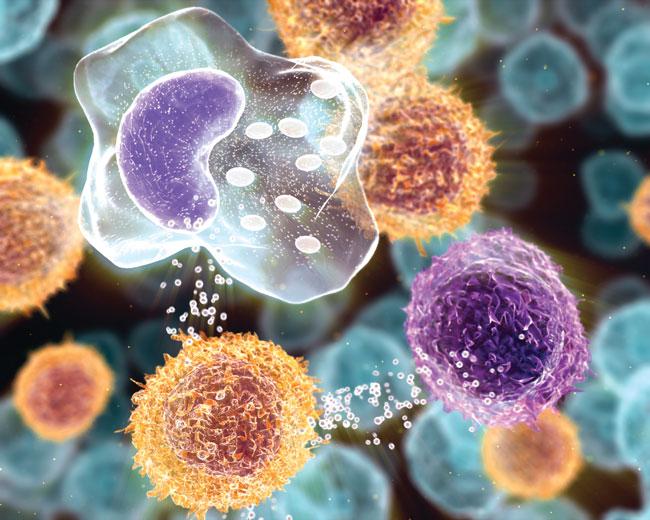
Inflammation’s Role in Disease
Realm of Imagination: How is it possible for the body’s system for initiating healing to also trigger harm? Could the inflammatory response, so recognizable when rallying to subdue the itch of an insect bite or to heal the cellular damage in a stubbed toe, also be a quiet instigator of diabetes, neurodegenerative disease, perhaps even cancer?
From Shadow to Substance: Immunology has always been a diverse area of study, yet the full scope of inflammation’s role in the body has taken time to reveal itself. “Immunologists increasingly appreciate that inflammation touches many physiological systems,” says Diane Mathis, an HMS professor of pathology. First was the discovery of its contribution to cardiovascular and metabolic disorders such as atherosclerosis and type 2 diabetes, followed by its links to multiple sclerosis, cardiovascular disease, and neurodegenerative diseases. Recently, interest has sharpened over whether inflammation, together with cigarette smoking and obesity, triggers some cancers.
The Harvard Dimension: Research at HMS has expanded greatly our understanding of inflammation’s involvement in disease, says Mathis. Bruce Spiegelman, the Stanley J. Korsmeyer Professor of Cell Biology and Medicine at HMS, was among the first to recognize that chronic, low-grade inflammation is a link between obesity and type 2 diabetes, showing that fat cells produce mediators typically found under inflammatory conditions. Research led by Steven Shoelson, an HMS professor of medicine, has suggested that an aspirin derivative may quell this inflammation in humans with diabetes.
What’s Next? “Two words will be key in the future of immunology-based therapies: natural and selective,” says Mathis. “We’ll discover more of the cells and molecules that participate in the body’s inflammatory response and learn how to use and enhance them to inhibit disease-related inflammation. We’ll also discover how to selectively deliver those inhibitors so that we can stem inflammation where it is harmful without compromising its beneficial roles in wound healing and infection fighting.”
Preimplantation Genetics
Realm of Imagination: Even if a baby’s health could be scripted, should it be? If screening newborns for a troubling metabolic condition helps prevent mental retardation, shouldn’t screening a fertilized egg’s genome for incurable conditions be a gift to would-be parents worried about the risks of lifelong disease or disorder?
From Shadow to Substance: Until relatively recently, the idea that you could learn the information contained in your genome bordered on the unthinkable. Even more extraordinary, says Cynthia Morton, the William Lambert Richardson Professor of Obstetrics, Gynecology and Reproductive Biology and professor of pathology at Brigham and Women’s Hospital, was the idea that such information could be commercially obtained. But with direct-to-consumer groups providing on-demand genetic testing and the cost of genome sequencing dropping —from $3 billion in 2003, to projections of $1,000 by 2014—Morton adds, the field is off and running.
The Harvard Dimension: The Personal Genetics Education Project, an effort directed by Ting Wu, an HMS professor of genetics, addresses the social and ethical issues of personal genetics through interactive workshops for high school students. Those same social and ethical issues are core to the Genomic Medicine Leadership Program, which Morton helped spur at Brigham and Women’s. This program aims to prepare primary care doctors for their patient’s questions; a key component of that preparation comes when the participants receive their own genetic profiles.
What’s Next? “We will develop more sophisticated computer algorithms to help us interpret which genetic variants cause disease,” says Morton. “This is not a trivial undertaking; we need this information to answer the questions people will have. I could imagine, for instance, a Facebook group for people who share a mitochondrial haplotype, one relating them to some woman who lived 30,000 years ago in the Caucasus, perhaps. And I expect that soon every newborn screen will include a genome sequence.”
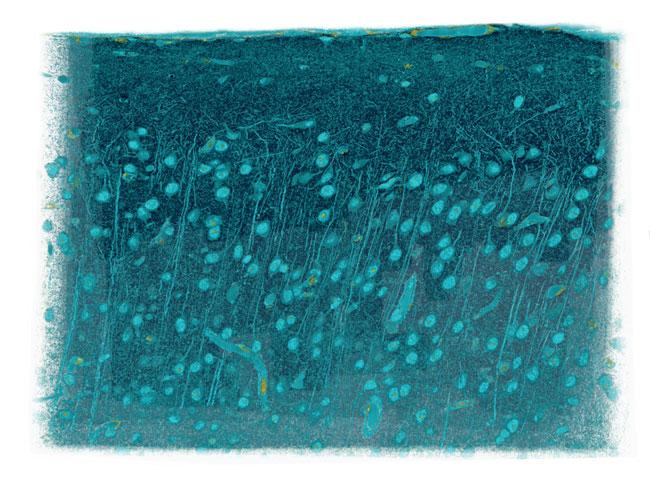
Neurons Made Visible
Realm of Imagination: What if we could make visible the workings of the brain? What understanding of neurological function might develop if we could watch the brain’s millions of neurons in action and trace the thousands of connections each make?
From Shadow to Substance: Our knowledge of what neurons do began not with sight, but with sound. In the 1960s, Nobel Prize recipients David Hubel, the John Enders University Professor of Neurobiology Emeritus at HMS, and his former HMS colleague Torsten Wiesel placed electrodes within a living mammalian brain and listened to neurons at work. Groundbreaking as this was, it was like “listening in the dark,” says Clay Reid, an HMS professor of neurobiology. “You had no idea what the neuron you were eavesdropping on looked like.” The chance to glimpse busy neurons improved with the advent of the two-photon microscope, a laser-based scanning microscope, and the creation of fluorescent molecules that brighten when neurons are active.
The Harvard Dimension: In 2002, Reid first used the two-photon microscope at HMS to view neural activity in the living brain. Since then, the list of neurobiology colleagues who regularly use the tool has grown: professors Wade Regehr, David Corey, and Bernardo Sabatini; associate professor Rachel Wilson; and assistant professor Robert Datta all use the two-photon microscope in their research, for example. In 2009, Sabatini customized the tool, bringing into focus the details of synapses, structures through which neurons talk to each other, as well as of dendritic spines, lollipop-like structures that support the synapses.
What’s Next? “High-end light microscopy will develop as a field,” says Reid. “We will be able to gather astonishingly large data sets from the functioning brain. We’ll capture images of entire neural circuits to see many thousands of neurons doing their job in a living brain, and, with electron microscopy, we’ll then study the connections between these cells. Brain circuits in all their complexity will finally be visible.”
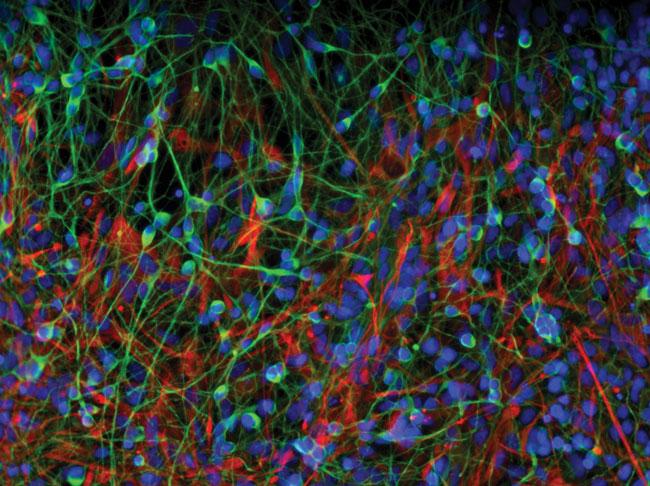
Reprogrammable Adult Stem Cells
Realm of Imagination: What if the body’s cells could be instructed to treat disease or repair damage? The notion of healing at the cellular level has long tantalized researchers. Embryonic stem cells brought promise—then pitfalls. What was needed was a readily accessible supply of adult cells that, like their embryonic progenitors, were willing to assume new roles.
From Shadow to Substance: Building upon the knowledge that nearly all cells harbor the same genetic code, researchers have searched for ways to reprogram differentiated adult cells. In 2006, they discovered key reprogramming tools: a mere four molecular factors that could take differentiated cells, strip them of their adult identities, and then coax them to develop into new cell types—converting skin cells into liver cells, for example. These induced pluripotent stem, or iPS, cells rocked the world of medical research. “It was a huge, unanticipated breakthrough,” says George Daley, an HMS professor of biological chemistry and molecular pharmacology. “The idea that we could turn specialized cells back into embryonic stem cells had been the stuff of science fiction.”
The Harvard Dimension: The news that such a small set of factors could alter the fates of cells was met with some skepticism, says Daley. But Konrad Hochedlinger, an HMS assistant professor of medicine, refined the technique, and Daley’s lab showed it worked in human cells. Daley’s group has also built a large repository of disease-specific cells that can be used to test therapies. Other Harvard researchers are expanding the list of cells that can be reprogrammed and are developing new methods for making cells reprogrammable.
What’s Next? “There are so many things going on in stem cell research,” says Daley, “that it wouldn’t surprise me if in another ten years we achieve something momentous—regenerating an organ, like a heart or liver, or tissue, like bone marrow, which is what we’re trying to develop in my lab. And along the way, we might be able to correct any genetic defects in those repurposed cells.”
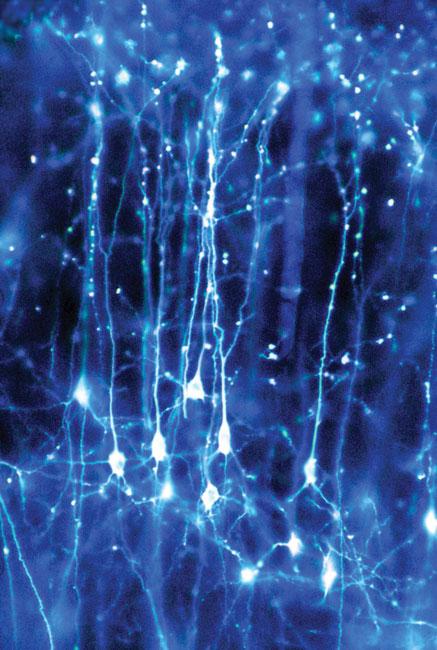
The Underpinnings of Neurodegeneration
Realm of Imagination: Why do the plaques and tangles of Alzheimer’s disease appear in the brain’s connection pathways and not also in its sensory, motor, or primary speech centers? And why are the dopamine-killing lesions of Parkinson’s disease only found deep within the brain’s movement-control center?
From Shadow to Substance: The puzzlement surrounding Alzheimer’s and Parkinson’s has been clearing as researchers identify proteins key to the development of these diseases. Their discoveries have also taught us more about the brain. “One lesson,” says Joseph Martin, the Edward R. and Anne G. Lefler Professor of Neurobiology at HMS, “is that the brain is never ‘off.’” Even when we sleep, the brain is at work. Especially busy are its connection pathways. Recent studies have shown that these pathways are focal points for aggregation of the protein amyloid beta. As a person develops Alzheimer’s, amyloid beta gums up in synapses, causing neuron-to-neuron connections—and the ability to form memories—to break down. Other work has advanced our knowledge of the genetic basis of neurodegeneration by finding that a gene defect in some people with Parkinson’s leads to the production of the protein alpha-synuclein, the agent that kills dopamine-producing brain cells.
The Harvard Dimension: “The best collaborative efforts at HMS,” says Martin, “are those between a basic science researcher and a clinician in a hospital laboratory.” A recent collaboration between neurobiology professor Bernardo Sabatini and Dennis Selkoe, the Vincent and Stella Coates Professor of Neurologic Diseases at Brigham and Women’s Hospital, identified how amyloid beta interferes with synaptic modulation of memory circuits. The Harvard NeuroDiscovery Center, another collaborative effort, aims to understand and find treatments for Alzheimer’s, Parkinson’s, and other neurodegenerative diseases by pooling Harvard-based expertise.
What’s Next? “Neurodegenerative disease research is at a great stage in its development, similar to where research on heart diseases was 30 years ago,” says Martin. “Genetic pathways will help us identify specific targeted molecular drugs for these diseases.”
Regenerative Cells
Realm of Imagination: When a person’s heart, kidney, or lung falters, physicians largely rely on the organ to heal itself, or drugs to boost its function, or transplantation to give new hope. But each carries risks. Wouldn’t it be best if organs could reliably repair themselves? Wouldn’t it be grand if medicine could harness the body’s potential for regeneration?
From Shadow to Substance: Working with induced pluripotent stem cells, Kenneth Chien, the Charles Addison and Elizabeth Ann Sanders Professor of Basic Science at HMS, has sought to make tissue that mends the muscle of failing hearts. About five years ago, he startled the field of regenerative medicine by discovering a progenitor cell that could generate functioning strips of mature heart muscle tissue. Then he identified a “master” cardiovascular stem cell, one that gives rise to cardiac, smooth muscle, and endothelial heart cells; may help form heart muscle and coronary arteries; and may play a role in the heart’s pacemaker system. The value of such a cell is enormous; it holds the potential for uncovering the origins of the human heart as well as for new therapies that repair, replace, or renew damaged tissue.
The Harvard Dimension: One leader in the field, Douglas Melton, co-chair of the Department of Stem Cell and Regenerative Biology at HMS and co-director of the Harvard Stem Cell Institute, provided early inspiration when he generated specific cell types from stem cells. Breakthroughs like Melton’s, Chien says, allow investigators to “envision the power that systems for redirecting cell development can have, from their use in modeling human disease, to the most elusive goal of all—personalized regenerative medicine.”
What’s Next? “Regenerative medicine is between acts, in an intermezzo period,” says Chien. “We’re moving from stem cell biology toward stem cell therapeutics. We’ll no longer just insert cells into the heart and hope they go where we want and do what we want. Instead, I think we’ll use pluripotent stem cells to make functioning ‘heart parts’ that we can simply put into place.”
A New Generation of Antibiotics
Realm of Imagination: Is it possible to stem the population’s growing resistance to antibiotics, to rescue the healing power of these medical workhorses? Does the door to discovery hinge only on finding new, broadly targeted bacteria-fighting agents or does it also open on ways to equip antibiotics with new tools to dismantle old foes?
From Shadow to Substance: “In the golden age of antibiotics,” says Suzanne Walker, an HMS professor of microbiology and molecular genetics, “everyone wanted a broad-spectrum antibiotic—and they were not difficult to find.” The deadly effectiveness of those drugs is waning, and scientists like Walker now search for new ways to fight pathogenic bacteria. Increasingly, investigators seek points of weakness in individual species, such as Staphylococcusaureusand other “superbugs” that defy treatments. Walker’s research has shown how one molecule, wall teichoic acid, ensures that S. aureuscells divide properly and form bacterial cell walls strong enough to withstand hostile environments. Walker’s work may lead to modifications of potential antibiotics to interfere with bacterial cell wall assembly. Imaging advances have also assisted the search for novel ways to defeat pathogenic bacteria. Fluorescent imaging can now follow a bacterium as it lives and dies, revealing how and when antibiotics kill the invader by compromising cell membranes.
The Harvard Dimension: State-of-the-art facilities, such as the School’s Institute for Chemistry and Cell Biology, allow Walker and others to screen antibiotics for their ability to disrupt bacterial biosynthetic pathways. A federally funded project on antibiotic-resistant infections headed by Michael Gilmore, the Charles L. Schepens Professor of Ophthalmology at HMS, is focusing Harvard’s research talent on new ways to fight pathogenic bacteria.
What’s Next? “We will learn more about the life and death of bacteria,” says Walker, “so that we may exploit pathways key to bacterial survival. And we need to learn how to prevent bacteria from transmitting resistance.”
The Many Roles of RNA
Realm of Imagination: What happens when a research project becomes a prism through which researchers can view anew the fundamental cellular processes of transcription and translation? They might just find that RNA, long a comfortable character actor in that gene–protein drama, assumes many guises and may even hold a principal role.
From Shadow to Substance: The discovery of small RNAs likely resulted from such a prismatic view. These tiny molecules regulate the production of thousands of proteins in plants and animals. Pinpointing their roles was aided by new tools for sequencing large amounts of DNA. “With this technology,” says Gary Ruvkun, an HMS professor of genetics at Massachusetts General Hospital, “we could suddenly see all the genes of several organisms.” Ruvkun used the nematode Caenorhabditis elegansto isolate let7, one of the first microRNAs. Ruvkun found let7to be pivotal to C. elegans’ development; he also discovered it within the genomes of other organisms. This clue meant that small RNAs were conserved over eons and species, making the molecules candidates for treating gene-based diseases.
The Harvard Dimension: Research on small RNAs has benefited a large fraction of studies at HMS, says Ruvkun. Clinical research by Judy Lieberman ’81, an HMS professor of pediatrics at Children’s Hospital Boston, found RNA interference, or RNAi, disabled genes that transmit the herpes simplex virus type 2. Other work, co-led by Louis Kunkel, also an HMS professor of pediatrics at Children’s, looks to microRNAs to treat muscular disorders.
What’s Next? “Small RNA research has two great thrusts,” says Ruvkun. “One is its use as a technical tool, and the other is its interesting biology. Many researchers use RNA as a tool, and their work is revolutionizing how laboratory biology is done. But the real revolution will occur when scientists discover why RNAi works so well, what processes it’s mediating, and what it’s doing in organisms. These investigations eventually will lead to the successful use of RNAi in medicine.”
Images: Courtesy of Joanna Aizenberg, Wyss Institute for Biologically Inspired Engineering at Harvard University (top); F. Hoffmann-LaRoche LTD. (pathogens); Courtesy of Clay Reid, David Bock, and Wei-Chung Lee (blue cube); Courtesy of Rekesh Karmacharya/Broad Institute and McLean Hospital (filaments); Dr. Jonathan Clarke/Wellcome Images (neurons)