Decoding the Chemistry of the Microbiome
Scientists zoom in on microbial messages at the molecular level to understand how the microbiome shapes health
Winter 2025
- 9 min read
- Feature
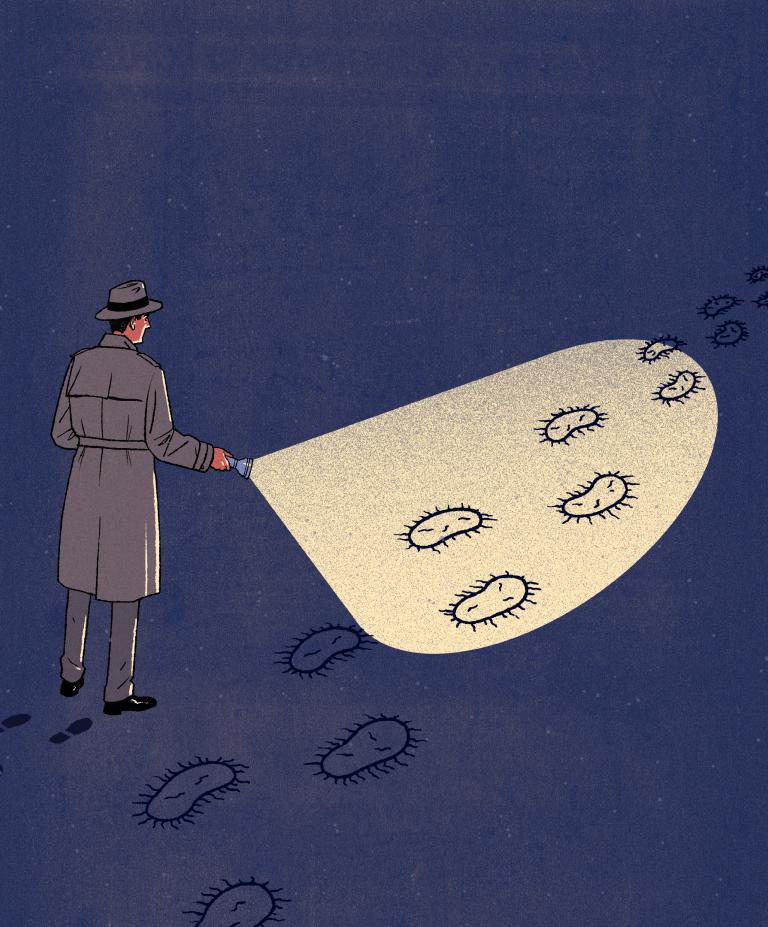
Each human body is home to trillions of microbes, whose combined cells may outnumber human cells. There’s no question that these microbiomes — the ecosystems of bacteria, fungi, and other microbes residing on and inside us — shape our health. But how, exactly, do they do it?
It’s a question that captivated Sloan Devlin when she was a young researcher fresh out of a PhD program in organic chemistry. It was the early 2010s, and Devlin had seen the many studies and headlines linking the microbiome with everything from autoimmune diseases to antibiotic resistance, metabolism, and even mood. But research explaining why those links existed seemed vanishingly sparse. That knowledge gap didn’t faze the budding scientist; instead, she says, it “seemed like a breath of fresh air.” She’d been searching for a way to put her chemistry knowledge to use to tackle big, unanswered questions in biology.
More than a decade later, she admits that the thought was rather naive. “There are huge challenges that come with entering a field that is so complex,” wrote Devlin, now an associate professor of biological chemistry and molecular pharmacology in the Blavatnik Institute at HMS, in 2022. “How do we begin to dissect the effects that hundreds of species of human-associated bacteria making thousands of molecules have on multifaceted diseases?”
It’s still an open question. But as more chemists like Devlin enter the field, they’ve helped propel microbiome research into a new phase: from raising suspicions that the microbial ecosystems within us profoundly influence our health to understanding the mechanisms through which they do it. What they’re discovering will be key to developing therapies that target the microbiome to improve health.
Cause and effect
When Devlin was starting her career, most microbiome research was descriptive: Studies showed correlations between the microbiome and health but not necessarily causation. Progress in DNA sequencing technology in the 1990s and early 2000s had made it much easier for scientists to figure out which microbes were inhabiting a person’s gut or other parts of the body. Researchers found obvious patterns in the microbiome composition of people with particular diseases versus healthy controls. “But if you stop there, it’s like stamp collecting,” Devlin says. “It doesn’t tell you what the microbes do.”
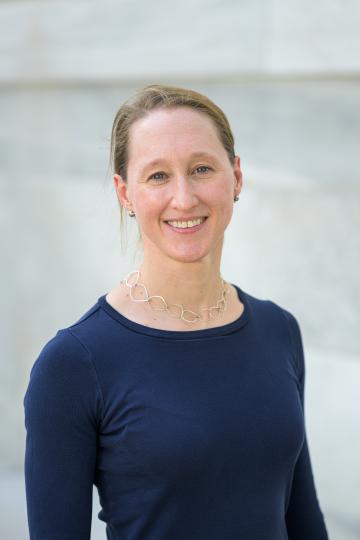
More causative clues emerged when researchers began manipulating microbiomes to change phenotypes (observable traits, such as disease symptoms). Scientists would find a mouse model of fatty liver disease, for example, and then treat the mouse with antibiotics to erase its microbiome. If erasing the microbiome changed the liver disease or cured it, that suggested the microbiome was somehow involved.
Researchers also experimented with mice that were raised “germ-free,” meaning they weren’t exposed to any microbes that could colonize their bodies. They started performing a kind of microbiome transplant, transferring fecal samples from other mice or humans with a given disease to the germ-free animal. “If the animal acquires the phenotype, that means there’s something the microbiome is doing that is contributing to that phenotype,” Devlin says. For example, studies in the early 2000s found that these transplants could transfer traits such as obesity and insulin resistance from humans to mice.
These types of studies, which are still common today, have started to tease out causative relationships between the microbiome and health. But they aren’t quite specific enough to be translated into treatments. Fecal transplants, for instance, typically include thousands of bacterial strains, all of which interact and play different roles, complicating any effort to pinpoint specific causes.
“The really hard part is what happens next — finding a specific bacterial strain and specific molecules that cause the phenotype,” says Devlin. That’s the problem she took on when she landed a postdoctoral fellowship at the University of California San Francisco in the lab of Michael Fischbach, a Harvard PhD alumnus who had previously worked in the HMS lab of Christopher T. Walsh. Fischbach and Devlin started parsing exactly which small molecules bacteria were producing inside the human body and what those chemicals might be doing — an effort “that seemed unusual at the time,” recalls Fischbach, who is now a professor of bioengineering at Stanford University.
In retrospect, though, the contributions of researchers like Devlin and Fischbach were needed. “In order to figure out what molecules are there,” Devlin says, “you need chemists.”
Good chemistry
The interactions between microbes and the human body often come down to specific chemicals — and the microbiome, particularly in the gut, is a massive, mysterious chemical gold mine.
Fischbach attributes this chemical diversity to the fact that the microbes living in our intestines have a tough job: to produce energy, they need to find electrons, extract energy from them, and dump them somewhere — all without access to oxygen that would make the process more efficient. This anaerobic metabolism “explains a lot of the artisanal chemistry you find among bacteria that live in the gut,” he adds. “We’re all living in their bus exhaust.”
That exhaust includes a rich and diverse array of molecules that microbes metabolize from the food we eat and the chemicals our bodies produce: short-chain fatty acids, amino acids, vitamins, peptides, lipids, steroids, and more. Many we simply tolerate or excrete. But after coevolving with resident microbes over millions of years, human bodies may have also learned to harness or interpret their chemical signals: to get a snapshot of the intestine, for example, so we know when to be hungry and when to be sated, or an immunological update regarding “who’s there and whether they pose a threat,” Fischbach says. Perhaps health problems emerge when this balance is interrupted. The ecosystem changes, the chemistry gets thrown off, and signals get jumbled.
Microbes have another important goal besides energy production: They have to stake out their territory in the body. That may be why some have evolved the ability to manufacture molecules that maintain the health of the intestinal lining and tamp down immunity — basically telling the host’s immune system, “don’t kill me,” Devlin says. Other microbes might release chemicals that help them compete, like toxins that poison competitors or antibiotic compounds that inhibit others’ growth.
Many of these small molecules cross into the bloodstream and circulate through the body, some in concentrations comparable to pharmaceutical drugs. That means each of us could have “thousands of unique chemicals that are of bacterial origin circulating, at least some of which act as signals to human cells,” says Marco Jost, an assistant professor of microbiology in the Blavatnik Institute at HMS.
The catch is that the vast majority of these chemicals, and the responses they elicit, remain mostly unknown.
Mining for molecules
So, how do chemists figure out what these chemicals do? Some start with a microbe of interest that has been identified in correlative studies. Then they can use DNA analysis to explore that microbe’s biosynthetic gene clusters, groups of genes responsible for producing bioactive compounds like antibiotics, to see if there’s anything useful. Fischbach used that DNA-based technique early in his career, including when he and colleagues discovered a molecule with antibiotic properties called lactocillin, which is produced by Lactobacillus gasseri, a common bacterium of the vaginal microbiome.
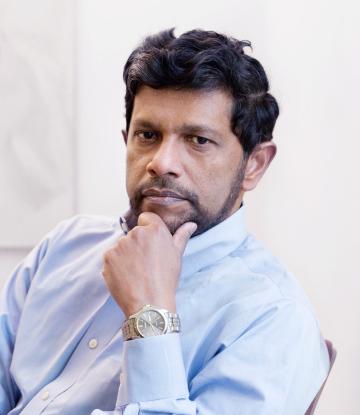
Another option is to “chew up the bacteria to see what molecules they can make,” says Ramnik Xavier, the HMS Kurt J. Isselbacher Professor of Medicine in the Field of Gastroenterology at Massachusetts General Hospital. A practicing gastroenterologist, Xavier follows patients with gastrointestinal conditions such as Crohn’s disease or ulcerative colitis to understand how their microbiomes change as those diseases emerge and flare.
Xavier and colleagues take several different approaches to untangle causality in the microbiome. One method involves taking samples of suspicious gut bacteria from study participants, growing them in the lab, and then partnering with colleagues who specialize in chemistry, such as Jon Clardy, the Christopher T. Walsh Professor of Biological Chemistry and Molecular Pharmacology in the Blavatnik Institute at HMS. Researchers in Clardy’s lab use a technique called activity-guided fractionation to break down the microbes’ bodies into individual components like cell wall fragments, lipids, and soluble molecules. Then Xavier’s team can expose each of these components to human cells to see how they might trigger immune reactions or have therapeutic effects.
For example, Xavier and colleagues had previously found dramatic links between a bacterium called Ruminococcus gnavus and Crohn’s disease; as patients’ R. gnavus levels increased from making up less than 1 percent of their gut microbiota to more than 50 percent, their autoimmune disease flared. But it was unclear why. So, Xavier worked with Clardy and postdoctoral fellow Matthew Henke to grow colonies of the microbe and take stock of all the molecules it was making, ultimately figuring out that R. gnavus produces a polysaccharide that antagonizes the immune system.
Scientists can also probe the problem from the reverse direction: starting with molecules rather than microbes. Devlin and colleagues use an instrument called a mass spectrometer to compare the molecules found in fecal samples from patients with a disease to those found in people without the disease. That helps them identify the chemicals that seem to be increased or decreased in the presence of the condition. They examine the levels of those molecules in both germ-free mice and those colonized with microbiota to evaluate whether those molecules are dependent on the presence of the microbiome. Then they design experiments in animals to test whether those molecules of interest could have an effect.
Devlin says this approach, called metabolomics, is “strain agnostic” — they don’t need to worry about the microbes involved until after they establish that a molecule is contributing to or protecting against a disease. This is the route they took when discovering that a metabolite influenced by the microbiome called cholic acid-7-sulfate, which reduces glucose intolerance in obese mice, is increased after bariatric surgery. In another study, they found that a bacterium makes a molecule called cholesterol sulfate, which inhibits immune cell migration, tamping down immune responses in the host.
Researchers in Jost’s lab are experimenting with new methods to determine how the human body senses and reacts to molecules produced in the microbiome. They use datasets from metabolomic studies to identify molecules of interest, which they then add to organoids — tiny, lab-grown clusters of cells derived from human cells that mimic the structure and function of tissues and organs. The researchers use CRISPR-based methods to home in on key pathways and receptors in the human cells, adding or removing genes from the cells to test whether responses to the signals are affected.
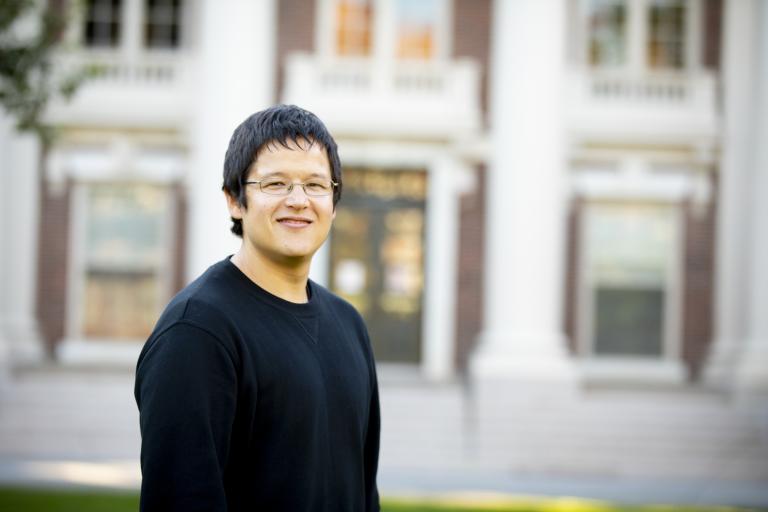
Jost says that chemists at Harvard have already “done really deep work figuring out which chemicals microbes make and by what mechanisms. What we bring is a complementary perspective to further interrogate what these molecules do.” Collaboration among a diverse group of scientists — immunologists, neuroscientists, cell biologists, physiologists, and others — is what he appreciates most about researching the microbiome. “People from their own disciplines are realizing that the microbiome plays a role in the processes they’re interested in,” he says. “As everyone brings their perspective, it makes for some cool science.”
A volatile mix
These types of collaborations with chemists are “paying off big time,” says Xavier, who is also a core institute member at the Broad Institute of MIT and Harvard. “We’ve been able to identify an amazing collection of molecules.”
In some recent studies, scientists at HMS have connected a single microbe to a molecule it makes to a pathway it operates through all at once, a rare feat in the field of microbiome research. For example, a 2022 study from Xavier and Clardy found that a lipid produced in the cell membrane of gut bacterium Akkermansia muciniphila could communicate with receptors on the surface of immune cells to trigger the release of certain cytokines, helping to explain why the bacterium has been found to protect against inflammation and improve response to cancer therapies. And researcher Megan McCurry in Devlin’s lab recently discovered that the gut bacteria Gordonibacter pamelaeae and Eggerthella lenta gobble up steroids found in bile and transform them into sex hormones and neurosteroids — including, surprisingly, a natural product called allopregnanolone that is the first FDA-approved drug for postpartum depression.
Devlin sees her research as an avenue for more precise molecule discovery. “If we in the lab could discover a small molecule that’s beneficial, then a pharmaceutical company could take up that idea and use it as a starting point, and make a drug out of it,” she says. Alternatively, if a detrimental molecule were discovered, the goal would be to prevent bacteria from making it in the first place. That might involve microbiome manipulation but in a much more targeted form than something like an antibiotic. “We shouldn’t be in the business of killing off bugs if we don’t have to,” she says.
Treatments like these might require some patience in a young field where an understanding of mechanisms is only starting to emerge. “Figuring out the story of one molecule and what it does, what it contributes to physiology and disease, can be a decades-long quest,” says Fischbach. It took scientists that long to understand many of the other molecules important in medicine, from neurotransmitters like serotonin to lipids like cholesterol, in enough detail to harness them for therapeutics.
“In order to understand what the microbiome is doing to the host, we do need to get into a similar level of detail,” he adds. “Pioneering work from people like Sloan is beginning to make real progress.”
Molly McDonough is the associate editor of Harvard Medicine magazine.
Images: Jori Bolton (illustration); courtesy of Sloan Devlin (Devlin); courtesy of Ramnik Xavier (Xavier); Rose Lincoln (Jost)
Research at Harvard Medical School hangs in the balance due to the government’s decision to terminate large numbers of federally funded grants and contracts across Harvard University.