A Half-Century Quest to Explain Dyslexia
Albert Galaburda discusses brain clues, neurodiversity, and what mouse models can reveal about reading challenges in humans
December 2023
Albert Galaburda
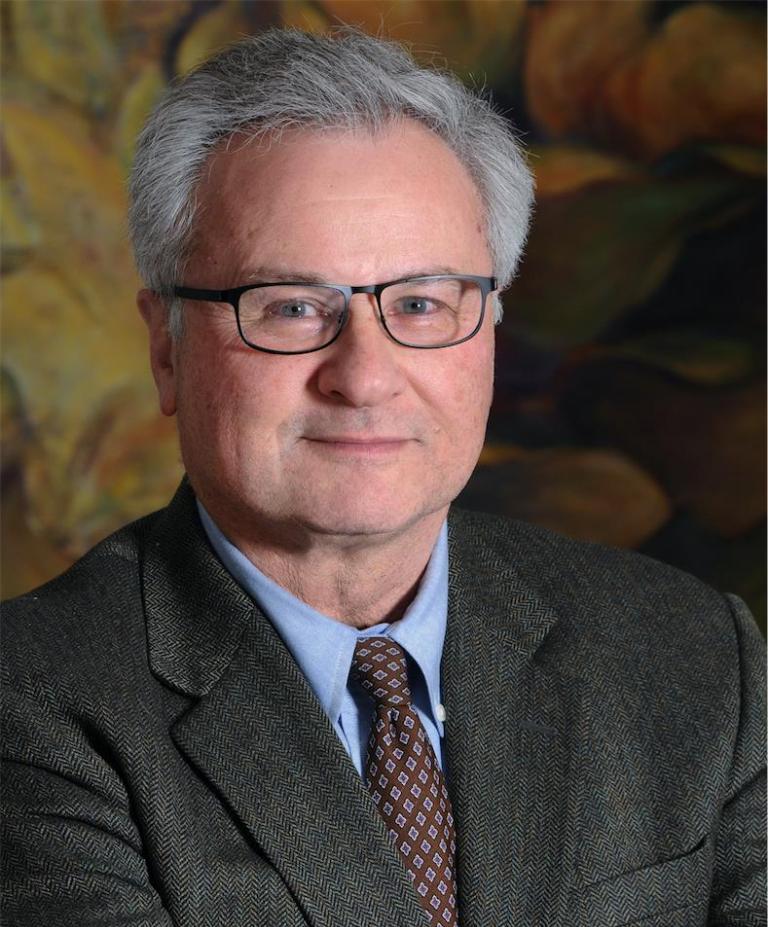
Albert Galaburda
For decades, researching dyslexia has been a passion and a fascination for Albert Galaburda, the Emily Fisher Landau Professor of Neurology, Emeritus, at HMS. Ask him why, and he’ll likely say that, for him, it is a quest to untangle a condition shaped by so many facets of who we are.
“It extends from sociology to molecular biology,” he says. “It has to do with how genes regulate themselves, but also with our brains, our schools, our education system, and our cultural attitudes toward reading.”
Prevailing views on dyslexia — which is marked by trouble processing written words — have evolved considerably over Galaburda’s fifty-year career. In the 1970s, Galaburda was among the first researchers to seek evidence of dyslexia in the brain. Along with colleague Norman Geschwind, MD ’51, he developed the Geschwind-Galaburda hypothesis, a foundational theory suggesting that varying patterns of early brain development — such as the asymmetry of brain hemispheres and the ways that neurons migrate — can affect cognitive abilities, including those associated with reading.
Geschwind and Galaburda’s early findings have shaped subsequent research in the field. Their work has shifted the thinking around dyslexia as a problem of laziness, vision, or poor instruction to one with neurobiological roots. It also has pushed scientists to understand how dyslexia can be shaped by both biological and societal forces. This understanding has provoked collaborations between neuroscience, psychology, genetics, and education that have been essential to developing early and effective interventions.
Galaburda spoke with Harvard Medicine about his career. An edited version of that conversation follows.
You spent much of your fifty-year career researching dyslexia. How did you get interested in it?
Originally I didn’t know anything about dyslexia. I was a neurology resident interested in language. During one study, we used a postmortem brain from a person with dyslexia to test one of our ideas: that language areas of the brain related to reading — the biggest task you can give a language system — were small and not well developed in people with dyslexia. But we actually found that those areas were relatively large in the dyslexic brain. And I noticed something else. In areas of the cortex associated with language, clouds of brain cells were found to be out of place: their usual migration paths had not been taken.
We looked at five or six additional postmortem brains from people with dyslexia and basically confirmed these initial findings of abnormal neuronal migration. We decided to try and model this abberant migration in rodents to understand the biology of how the neurons got to be there. Most of my career was spent studying how these changes developed in the brain.
But if written language is a human innovation, what could rodent brains tell us about dyslexia?
In the later part of my career, people had discovered genes that imparted a risk for developing dyslexia, and we were able to manipulate those genes in mice — to turn them on and off. We produced anomalies in the mouse brains, such as abnormal neuronal migration, that we had seen in the brains of people with dyslexia. This was the first demonstration of risk genes for dyslexia being associated with abnormalities in brain development.
We then gave the animals behavioral tests. We looked at acoustic processing, which was found to be abnormal. The affected animals could not perceive small bits of sound. The innovation of reading spurred this trait to adopt a new biological function without evolutionary modification, a phenomenon that’s called preadaptation.
In order to read, you have to map a bit of sound, called a phoneme, to a bit of text, known as a grapheme, so the ability to distinguish small bits of sound is crucial. The sound of the letter “p,” for example, differs by only 15 milliseconds from the sound of the letter “b.” If the hearing system is slow, the distinction can’t be made, and the letters are confused. This ability to distinguish sounds is particularly important when a person is learning to read; later in life people are able to guess at sounds that they can’t hear well.
We also found that these manipulations of the brain affected male rodents more than females, which was fascinating since dyslexia is more common in boys. We could trace these different responses between males and females all the way back to the brainstem, where sound first comes into the brain; the cochlear nucleus where the brainstem neurons process information from the ear.
In order to read, you have to map a bit of sound, called a phoneme, to a bit of text, known as a grapheme.
All of this led me, by the end of my career, to think that dyslexia was not a language problem initially, but instead a sensory issue related to perceiving sounds, one that leads to the development of abnormal phoneme representation in the brain. Not all sounds, but certain sounds that rapidly move. If you’re not getting the right phonetic representations in the brain because you can’t hear those sounds, you’re not able to map those phonetic representations onto letters or syllables. The cognitive system is intact; it just receives poor quality acoustic information early on, and the phonological disorder follows, This explains why a person with dyslexia can be very, very smart. It doesn’t affect cognitive ability.
One implication of this inability to perceive sounds is that, in principle, you could use some sort of hearing aid to slow down the signal coming into the ears of at-risk babies during the first year of life, when they’re forming those phonological representations. This might help prevent the sound perception problem.
Let’s talk about one idea you mentioned — that people with dyslexia can be very smart. Your colleague Norman Geschwind hypothesized that dyslexia may actually confer some sort of yet-unidentified evolutionary advantage. What do you think of that idea?
It’s estimated that 10 percent of people are dyslexic. It hasn’t disappeared from the population through natural selection, so we know it doesn’t confer something that prevents procreation. Dyslexia need not confer a superiority, and it will not become extinct so long as it doesn’t diminish survival.
Does the presence of something in the brain that is bad for reading cause it to reorganize in such a way that the brain is now good at other things?
The fact that you are dyslexic doesn’t guarantee that you’re going to be better at something else, but it often happens that way. Many people with dyslexia tend to be quite good with visual–spatial tasks. Many of them go into architecture or art or engineering. This brings up really interesting questions. For instance, do the special skills that some people with the condition show appear because they can’t read, so they’re going to spend a lot of time doing something else and get good at it? Or does the presence of something in the brain that is bad for reading cause it to reorganize in such a way that the brain is now good at other things? My guess is that it’s a bit of both. We know that in people who are born blind, parts of the brain dealing with vision now help with hearing and touch. Clearly, the brain can reorganize in dramatic ways.
Picasso was supposedly dyslexic. And so people have said, Hey, don’t muck with this because if you get rid of the dyslexia, you get rid of Picasso. Do we want to do that? And I agree, we have a lot to learn before we do anything like that. Either way, you can see that dyslexia is a human condition that spans a broad range of metaphors about who we are. We’re social beings; we’re molecular beings. It takes a lot of work to figure out what’s going on and how to help it without making things worse.
Molly McDonough is the associate editor of Harvard Medicine magazine.
Image courtesy of Albert Galaburda.