The Neuroscience of Cancer
Scientists are making surprising discoveries about the connections between cancer and the nervous system
- 11 min read
- Feature
Illustration: Dominic Kesterton
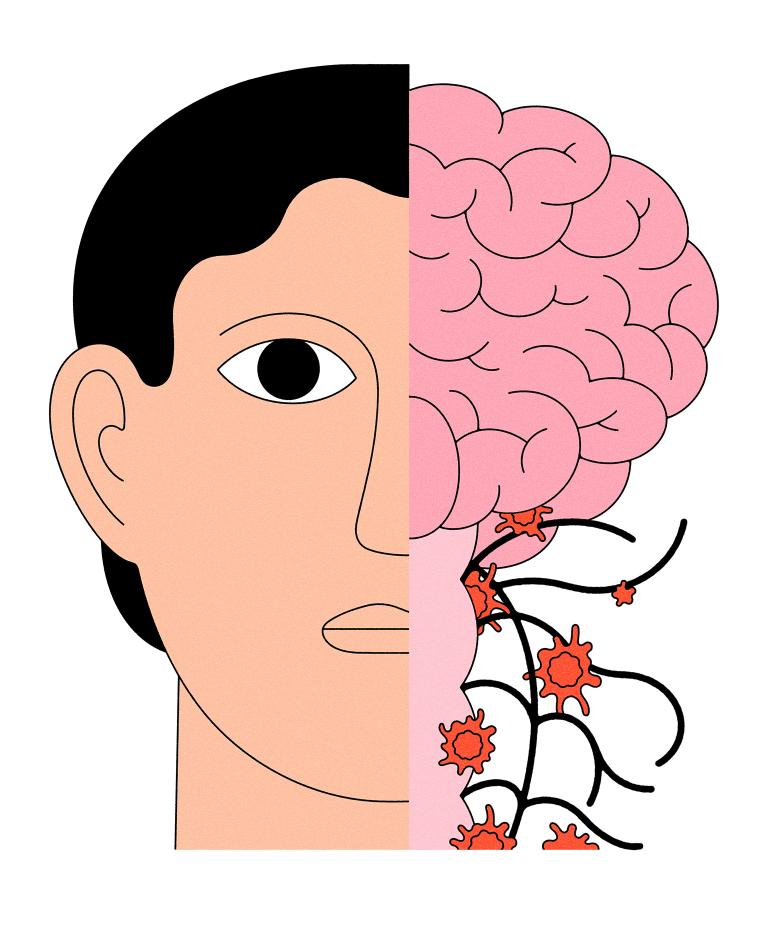
Illustration: Dominic Kesterton
Of the many tumor slides William Hwang, MD ’15 PhD ’13, has seen, there’s one in particular that is seared into his memory. He encountered it as a medical student while on a rotation caring for an elderly patient with pancreatic cancer. “What struck me as the most horrible part of the experience for them was the pain,” he recalls.
Hwang wanted to understand where that intractable pain was coming from. So, he asked Mari Mino-Kenudson, an HMS professor of pathology at Massachusetts General Hospital, to walk him through the patient’s tissue slides under a microscope. That’s when he saw a nerve encased within a tumor, being prodded and compressed by cancer cells. The patient was suffering from perineural invasion, a painful phenomenon in which malignant cells surround and invade nerves.
“I remember thinking that it looked like something out of a horror film,” says Hwang, who is now an HMS assistant professor of radiation oncology at Mass General. “I wondered why cancer cells would form such intimate connections with nerves. What do they derive from this relationship, and how can we intervene?”
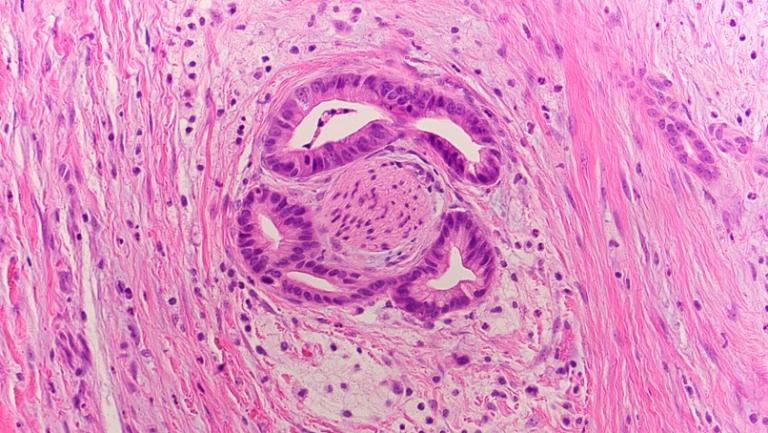
Hwang dug into the literature and found that perineural invasion was documented as early as 1897, when a urologist named Hugh Young used methylene blue staining to examine tumors and noticed something curious: bundles of nerve fibers creeping into many of his samples. Although nearly half of the tumors he examined showed connections with nerves, Young expressed surprise that no other researchers had yet investigated such links. “The question of the relation of nerves to tumors … is practically terra incognita,” he wrote in the Journal of Experimental Medicine.
It remained that way for nearly a century, with the presence of nerves in tumors passed off as coincidental. “If you look at all the components of a tumor, not everything is playing an active role,” says Hwang. “Some elements are just bystanders: wrong place, wrong time. Only more recently have people started to see the important biological connections.”
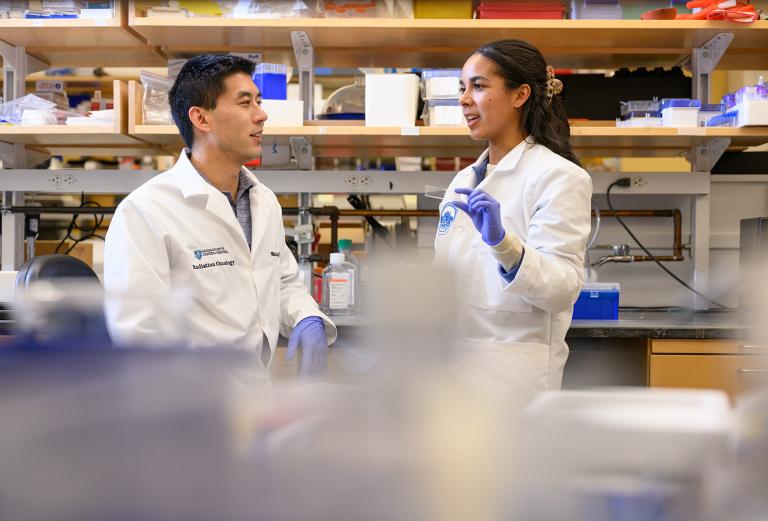
One of the first scientists to do so was pathologist Gustavo Ayala, who, in the early 2000s at Baylor College of Medicine, grew nerve and cancer cells together in lab dishes. The results were striking: Mouse-derived spinal nerve cells seemed to stretch out toward human prostate cancer cells, beckoning them. As the cells intermingled, the cancer cell colonies blossomed.
Around the same time, cancer researchers started appreciating the importance of the tumor microenvironment: the ecosystems of cells, molecules, and blood vessels that surround tumors. At the Albert Einstein College of Medicine, oncologist Claire Magnon and her team wondered what would happen to tumors in the absence of nearby nerves. When they grafted human tumors into mice and destroyed the sympathetic nerves around them, they found that the tumors stopped growing. Inhibiting the parasympathetic nervous system reduced the ability of tumors to spread and metastasize. Other researchers noted that a greater density of nerves around tumors in the prostate, breast, colon, and elsewhere could help to predict whether a tumor would be more likely to grow or recur. More recently, Hwang’s research involving patients with twelve types of cancer found that perineural invasion predicts worse outcomes.
Studies like these have fueled a growing realization that the nervous system may not be the innocent bystander in cancer that it was once considered to be. Instead, it likely plays a role in everything from tumor initiation and growth to metastasis and response to treatment. Perineural invasion is just one dimension of an emerging field, known as cancer neuroscience, that Hwang and a new generation of researchers are bringing into the spotlight. The communication signals they are discovering between cancer cells and nerves are opening up new possibilities for therapies that act on these links to treat the disease.
Yet cancer neuroscience “is still an early field, almost in its infant stages,” cautions Richard Wong, MD ’94, chief of head and neck surgery at Memorial Sloan Kettering Cancer Center. “People are only just starting to realize how important it is.”
Co-opted by cancer
Wong’s interest in cancer neuroscience is in part a matter of practicality. Since he started performing ear, nose, and throat surgeries in the 1990s during an otolaryngology residency at Massachusetts Eye and Ear, he’s been drawn to the “elegant” challenge of uprooting tumors while keeping nerves in the head and neck intact — nerves he describes as “critical to a person’s persona.” The invasion of nerves by cancers is a reality of his daily life. “Yesterday, I spent hours trying to peel a tumor off of a tiny nerve that enables a person to speak,” he says.
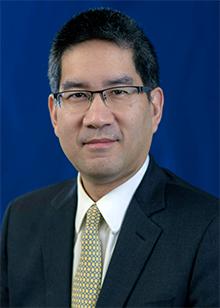
Such encounters are what first drew Wong’s attention to cancers that seem to track nerves, almost like the nerves are “highways for more extensive invasion,” he says. Around 2010, his lab at Memorial Sloan Kettering published one of the first studies to probe why this happens. The researchers studied interactions between nerve cells and pancreatic cancer cells in petri dishes and in mouse models and found that cancer cells migrated toward a protein called glial cell line–derived neurotrophic factor (GDNF) that is released by the nerves. This study showed that the nerves were actually inviting and enabling cancer spread.
Wong’s lab co-cultured cancer and nerve cells in petri dishes and took time-lapse videos, which showed cancer cells latching onto and moving along the nerves. That’s how Sylvie Deborde, a biologist in Wong’s lab, first noticed a mysterious, skinny cell darting between cancer and nerve cells, as if it were “grabbing and pulling the cancer cells toward the nerves, like pathfinders,” Wong says.
The researchers figured out that those skinny cells were Schwann cells. In a healthy peripheral nervous system, Schwann cells usually wrap around nerves and protect them; if a nerve gets injured, they “wake up” and get to work on repair. Wong and Deborde learned that these activated Schwann cells also attract cancer cells and create channels for the cancer to spread through. They’ve also found that pancreatic cancer patients with activated Schwann cells tend to have worse outcomes. “We believe that this Schwann cell behavior enables more aggressive cancer behavior,” Wong says.
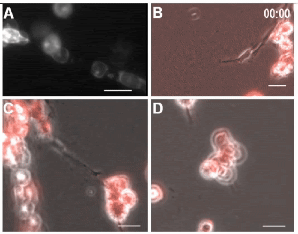
These findings hint at a wider trend: Cancer seems to have an uncanny ability to co-opt the nervous system’s own maintenance processes. “A theme we see repeatedly is that cancer is kind of like a traumatic event,” Wong says. “It destroys tissues around it and creates trauma in the area. And then it triggers and utilizes these innate programs in the host, in the environment, that try to repair the injury. But paradoxically, these repair mechanisms may actually promote cancer progression.”
Hwang’s research has revealed similar themes. For example, a 2021 study found that tumors with perineural invasion tend to express genes related to axonal guidance. These genes are typically activated when the nervous system is first developing, so the expression of the genes by tumors indicates that cancer cells could be hijacking normal growth processes to interact with and invade nerves.
A traditional approach to studying a tumor has been to blend its cells together and analyze the aggregate gene expression. But Hwang is instead using spatial transcriptomics — an approach that combines advanced imaging with RNA sequencing and complex algorithms analyzing massive data sets — to pinpoint the genes that are expressed within individual cells in different areas of a tumor.
“The areas of a tumor that feature interactions between cancer cells and nerves are often sparse, so if you don’t isolate them in space and analyze the tumor in its entirety, you’ll never pick up on the relevant signals,” he says. The methods that his group and other researchers have pioneered in spatial biology only really emerged in the last few years, but they now enable scientists to identify differences between tumor cells that are engaged with nerves and those that aren’t.

Hwang’s team recently used these spatial methods to uncover hints that pancreatic cancer cells spreading along and into nerves are more likely to express genes associated with a family of molecules called platelet-derived growth factor, or PDGF, which is usually involved in cell growth, tissue repair, and blood vessel development. By growing genetically engineered cancer cells with nerves in dishes, they confirmed that when cancer cells produce high levels of specific forms of PDGF, nerves are more likely to grow toward a tumor and help the cancer cells invade the nerves. They also found that targeting PDGF signaling may reduce perineural invasion, potentially improving outcomes — something that they are now actively testing in preclinical models.
While chipping away at the causes of perineural invasion is important, Wong says that the interactions he is studying in the peripheral nervous system are just a “small subset” of all the relationships scientists are exploring between cancer cells and nerves. Some of the most compelling nerve-cancer links are emerging in the central nervous system, where aggressive and deadly brain cancers grow in close proximity to dense networks of neurons and the cells that support them.
Electric findings
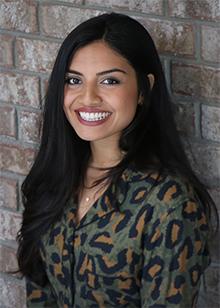
While working as a postdoctoral fellow in the Stanford laboratory of Michelle Monje in 2017, Humsa Venkatesh, now an HMS assistant professor of neurology at Brigham and Women’s, was exploring how neural activity might affect the growth of gliomas, the most common type of brain tumor. She had adopted an unconventional approach, taking tools traditionally used in neuroscience, such as optogenetics — using light to control the activity of genetically modified neurons to study and manipulate neural circuits — and applying them to study cancer.
By implanting cells from human gliomas into the brains of mice, Venkatesh was able to activate the mice’s brain cells and see how they were communicating. She vividly remembers the moment when she came to an important realization: Brain cancer cells were directly exchanging electrical signals with neurons. She became one of the first scientists to witness this phenomenon as she watched tumor cells pulsate with green light on a computer screen. Her work showed that the glioma cells were exchanging signals with neurons — and with one another — by forming a kind of synapse, similar to the way neurons communicate in healthy brains.
The surprising finding showed that cancer doesn’t only connect with the nervous system via chemical messages or third parties, like Schwann cells. It can also integrate into neural circuits more directly, and this electrical communication can help it grow. And it happens in a feedback loop: Venkatesh found that the more the neurons fired signals, the more the tumors grew; the more the tumors grew, the more excitable the surrounding neurons became.
Venkatesh also found that the increased electrical activity she’d induced in the mouse brains was stimulating the production of certain proteins, including one called neuroligin-3. That protein normally helps the brain with cell growth and communication between neurons, but it also helped glioma cells to grow. When her team prevented the production of neuroligin-3 in mice, the cancer stopped in its tracks.
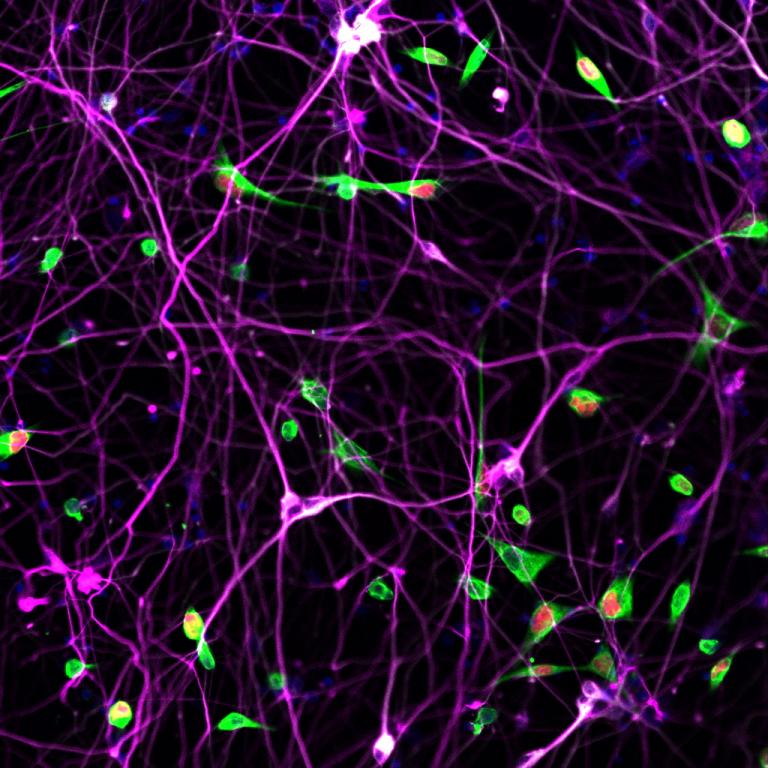
“We often find these proteins that are growth factors — they’re a dime a dozen,” she says. “But we were able to knock out just one of them and saw a complete stagnation of growth. It was unlike anything we ever see in cancer research.” A treatment based on these findings that suppresses production of neuroligin-3, led to a clinical trial.
Gliomas can be especially tricky to treat. Even after they are removed surgically, they tend to recur, sometimes in distant parts of the brain. In a study published in December 2024 from the labs of Bernardo Sabatini and Marcia Haigis in the Blavatnik Institute at HMS, neuro-oncologist Annie Hsieh helped to explain these recurrences by tracing the paths of neurons that form synapses with glioma cells. Using an injected rabies virus engineered to light up cells of interest in the brains of mice, Hsieh was able to capture images of all the glowing neurons leading to a glioma, providing visual proof that gliomas integrate into existing wiring patterns that extend across the brain.
These and other recent findings piggyback on Venkatesh’s research to illustrate that cancer’s synaptic connections with neurons are important and widespread, arising from different brain regions. “We’re not thinking about this from a local perspective, because of the way neurons work together. We know from neuroscience that they’re highly interconnected,” Venkatesh says.
That interconnectedness spreads to the peripheral nervous system as well. “Neural circuits are extensively connected throughout the whole body, from the brain to the periphery,” says Venkatesh. “So we’re trying to look at it from a systems perspective.”
Her lab has more recently been exploring whether cancers outside the brain with a high propensity to metastasize — such as skin, lung, and breast cancers — could be forming electrical connections with nerves similar to what she has seen in the brain, and whether that could help them travel to the central nervous system and grow there.
In a study that is currently undergoing peer review, for example, Venkatesh found that not only do connections with nearby nerves appear to encourage small cell lung cancer tumor formation in the lungs but also that the cancer cells can sense and respond to electrical signals from neurons. When the researchers exposed the cancer cells to neurons in the lab, the behavior and appearance of those cancer cells began to shift; some of them looked more like neurons that integrated into existing circuits, whereas others behaved more like neuron-supporting brain cells called astrocytes — indicating that neurons can help cancer cells adapt to the environment of the brain as cancer spreads.
Interactions and interventions
Although it’s taken time for the nervous system’s role in cancer to be appreciated, Venkatesh says the connections are logical. “The nervous system is so powerful in driving tumor growth, I think, because it is the master regulator of almost every process in the human body,” she says, “from the function of every organ to the function of every cell.”
That fact could complicate the development of treatments that target the crosstalk between cancer cells and nerves. “It’s difficult because the body has evolved these mechanisms over however many years to serve a purpose,” says Wong. “Maybe you can disable these Schwann cell repair mechanisms and slow cancer progression in a mouse. But if you disable them in a person, is that acceptable? Could it lead to problems with nerve function?”
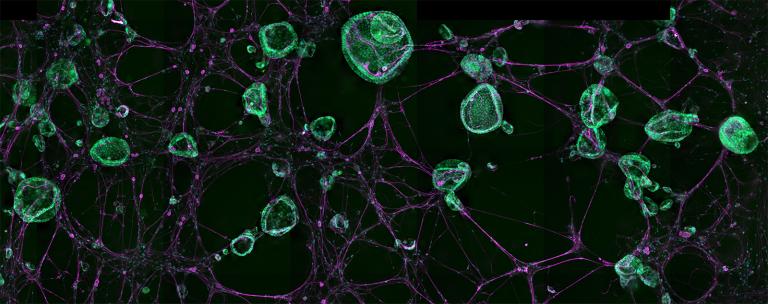
Wong says it will take time for researchers to overcome such hurdles. But he’s encouraged by the scientists entering the field who are willing to take risks and ask big questions. “In the past, it was clinicians like me who were interested in the relationship between nerves and cancer because we deal with them in the operating room,” he says. “But now there are a lot of young, talented scientists like Humsa and William who are making major discoveries and starting new labs.” As interest in cancer neuroscience grows, so does the range of questions being asked. Some researchers are exploring how cancer and nerve cells interact with the immune system, for example; others are focused on how chemotherapy affects cognition or how stress modulates tumor growth.
Scientists will need to decode many different specific neuron-to-malignant-cell interactions, which are likely to vary across the subclasses of neurons and branches of the nervous system — as well as the different organs and tissues where cancers grow. “It won’t be a one-size-fits-all mechanism,” Venkatesh says. “We need to be really smart about how we assess these interactions.”
But, she adds, because there are many different ways that tumors exploit existing mechanisms of neuroplasticity, there may be many different targets for treatment. There are likely also drugs that modulate neural circuits that have already gone through FDA approval — treatments for epilepsy, seizures, or depression, for instance — that could help thwart some of the interactions between cancer cells and nerves.
“What’s so interesting is that we’ve gotten a lot of clues about how these tumor cells will respond based on normal development, because they’re just rehashing the processes that already exist in the body,” Venkatesh says.
In other words, insights from neurology about how nervous systems help human bodies function, heal, and grow could inform interventions in oncology when those same processes promote cancer. “It’s this double-edged sword,” says Wong. “And it’s fascinating.”
Molly McDonough is the associate editor of Harvard Medicine magazine.
Research at Harvard Medical School hangs in the balance due to the government’s decision to terminate large numbers of federally funded grants and contracts across Harvard University.