Designing Brain-Computer Interfaces That Connect Neurons to the Digital World
Twenty years after meeting at HMS, two alumni are at the leading edge of efforts to use minimally invasive neural implants to improve human health
- 8 min read
- Feature
Cortex in Red, a depiction of the cerebral cortex by artist Greg Dunn

Cortex in Red, a depiction of the cerebral cortex by artist Greg Dunn
Craig Mermel, MD ’12 PhD ’10, was nervously waiting for his HMS entrance interview when a newspaper headline about the Mars Rover caught his eye. It was January 2004, and the spacecraft had just capped a seven-month voyage by landing on the red planet. The news sparked a conversation between Mermel and another jittery applicant — Ben Rapoport, MD ’13 — who was sitting nearby in the Dean’s lounge at Gordon Hall. “I remember thinking it was nice to have a distraction,” Mermel says. “Then we each got called into our interviews, and I thought, ‘Probably won’t see him again.’ ”
Mermel thought wrong. Twenty years after they were accepted to the Harvard/MIT MD-PhD program, the longtime collaborators are leading efforts to create brain-computer interfaces (BCIs) that connect neurons to the digital world. Modern electrode arrays can tune in to the activity of “hundreds or thousands of neurons at a time,” says Rapoport, now a neurosurgeon at Mount Sinai Hospital in New York City. By decoding how intentional signaling in the brain gives rise to corresponding physical movements, scientists can design systems that allow people to operate computers with their thoughts alone.
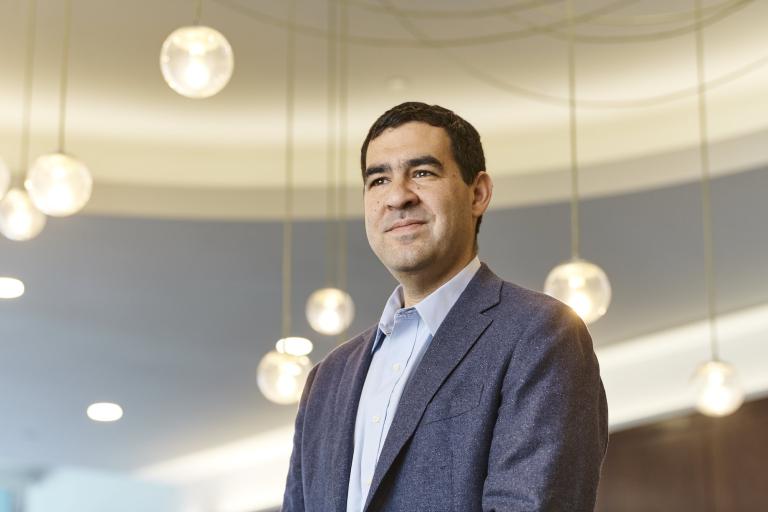
No longer confined to the realm of science fiction, BCIs are increasingly being tested in human studies. In 2021, Rapoport launched Precision Neuroscience, a BCI start-up with aspirations to restore lost functioning in patients with paralysis, and Mermel joined the company in 2022. BCIs have transformative potential for treating movement disorders. As of this writing, the company’s technology has been tested in eighteen patients, and Rapoport anticipates an early version of the technology could reach commercial markets in 2025.
BCI background
The term “brain computer interface” was coined in 1973 by Jacques Vidal, a computer scientist at the University of California, Los Angeles, who proposed that electrical signals in the brain might one day be used to control prosthetic devices. Researchers have since gone a long way toward realizing Vidal’s early vision. In 2003, a team at Duke University showed that monkeys implanted with microelectrode arrays could consciously control robotic arms. A year later, a young man named Matt Nagle became the first paralyzed person to benefit from BCI technology. A star athlete in his teens, Nagle had been injured during a knife attack that severed his spinal cord. But using a BCI, he could control a computer cursor and move a prosthetic hand.
The type of implant inserted into Nagle’s brain “marked a turning point for neuroscience and what became the BCI field,” Rapoport says. Called the Utah array, it was studded with up to one hundred needle-like electrodes that penetrate the brain’s outer layer. Electrodes used previously in neuroscience varied from one lab to the next. There wasn’t any standardized process for manufacturing the devices, “nor was there a standardized way of processing the signals,” Rapoport says. The development of Utah arrays changed that. Introduced in 1989, the devices were uniformly fabricated using the same methods used to produce microchips. Utah arrays have since been implanted in dozens of people and were adopted by BrainGate, a broad-based, multi-institutional effort that grew out of research at Brown University in the late 1990s and now includes researchers from HMS and Massachusetts General Hospital.
Converging advances in implant design and high-performance computing increasingly allow scientists to “translate the brain’s intrinsic, electrical language to something that’s intelligible by a human or a machine,” Rapoport says. That’s crucial to achieving the technology’s full potential, but it also introduces tricky ethical challenges, including “concerns over brain privacy,” says Gabriel Lázaro-Muñoz, an HMS assistant professor of psychiatry at Massachusetts General Hospital and member of the Center for Bioethics at HMS. Mermel agrees. Neural data belong to patients, he says, and should be used only in accordance with their consent and for their direct benefit. “This is an issue we are taking very seriously at Precision,” Mermel says.
Applied science
Rapoport and Mermel’s work in this field grew out of their time as students in the Harvard-MIT Program in Health Sciences and Technology (HST). The program has educational tracks leading to an MD from HMS and a PhD in medical engineering and medical physics from Harvard or MIT. Its mission is “not just to train good doctors but also to teach students to think about medicine as a place where there are broader problems to solve,” says Matthew Frosch, MD ’87 PhD ’87, the associate director of admissions for the HST program. Incoming students are anchored in rigorous science, Frosch says, “often with deep quantitative mathematics skills.”
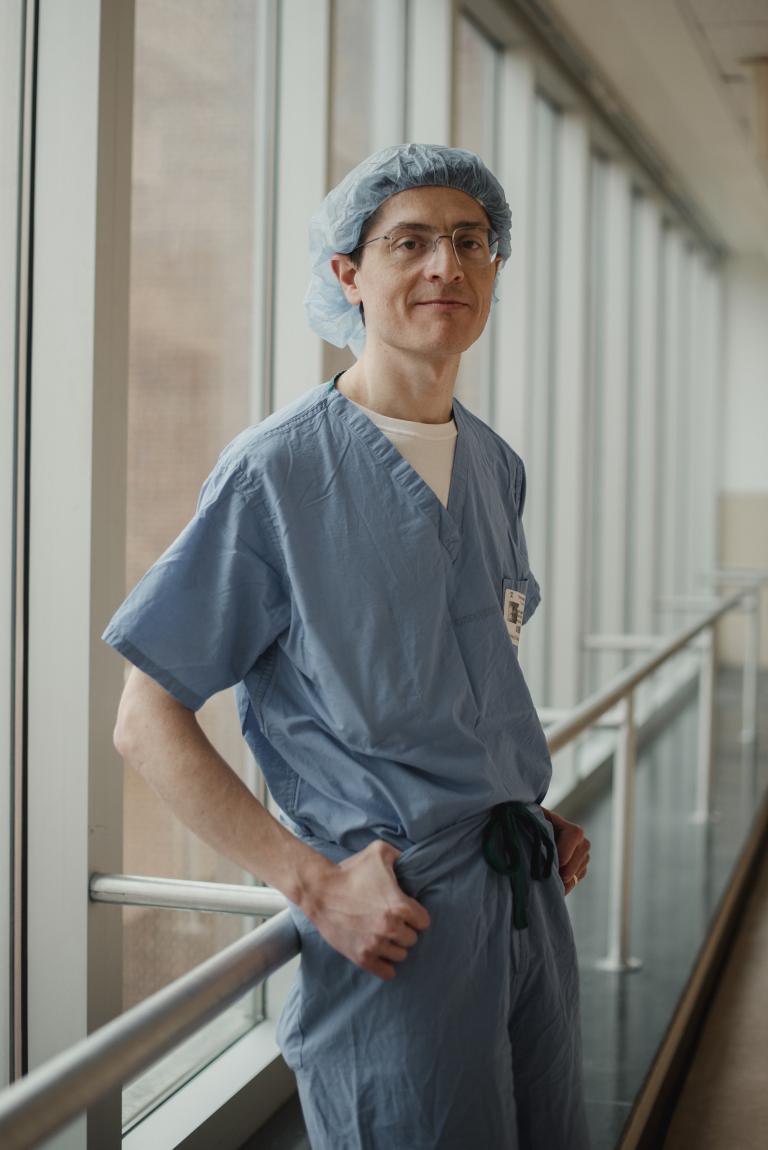
For Rapoport and Mermel, HST was an optimal fit. A math and biochemistry major during his undergraduate years, Mermel had a long-standing interest in the quantitative side of medicine. For his PhD research at Harvard, he developed computational tools for finding new drug targets in complex genomic datasets. Rapoport attended MIT for his doctorate, where he began his work on implantable electronics for brain chips — the subject that still motivates him today. The pair also honed their business skills during graduate school by creating a company that they subsequently sold to Apple. Called Simbionics, it was built around an analytics platform that used wearable sensor data to monitor cardiovascular fitness.
Mermel wound up taking a job at Apple after finishing his residency in pathology at Mass General. Rapoport, meanwhile, completed his residency and fellowships in neurosurgery at New York-Presbyterian/Weill Cornell Medical Center. He continued with his surgical career but was also recruited in 2016 to join Elon Musk and other scientists in cofounding Neuralink, a BCI start-up.
Neuralink developed an entirely new type of implant that contains more than a thousand electrodes distributed over polymer threads so thin they have to be stitched into the brain by a specialized robot. More electrodes mean more readings from individual cells, so compared to the Utah array, Neuralink’s implant affords a higher-bandwidth connection to the neuronal chatter. However, all penetrating electrodes share certain drawbacks. Scar tissue can form around them, diminishing signal quality over time, and moreover, “all penetrating electrodes damage the brain,” Rapoport says. “If you add more electrodes to scale bandwidth, then the damage increases. And if you’re doing that with a device that’s supposed to help vulnerable people — then there’s a bit of a paradox there.”
That paradox ultimately drove Rapoport to leave Neuralink in 2018 so he could pursue a different approach. The prevailing belief at the time was that penetrating electrodes were needed to achieve optimal signal strength. But Rapoport was encouraged by mounting evidence that high-quality readings could be obtained from the brain less invasively. Indeed, several other BCI companies have gone so far as to develop sensor-equipped helmets that measure neuronal signals from the surface of the scalp. Rapoport explains that conscious thought, movement, sensation, vision, and memory are all computed in the brain’s outermost layer, a thin mantle of tissue called the cortex. Whether electrodes read from within the neocortex or directly on top of it, “the distances are still very small,” he says. “And the surface activity is what we’re interested in.”
Rapoport soon teamed with Michael Mager, an investor and Harvard alum, to form Precision Neuroscience. And he asked Mermel, who was leading a team working on AI-assisted medical imaging at Google, to join as a board member. Mermel had little experience in either BCIs or neuroscience at the time. “My background was all in applications of data and machine learning to different types of health domains, and the practical matters of building products and getting them out into the world,” he says. But Mermel was intrigued by the prospect of using BCI technology to access very detailed information on cognitive processes such as movement and sensory processing. Treatments for neurological diseases had long been hampered by the lack of technology to extract high-quality data about the brain, he says, and “this is something that I saw companies like Precision helping to solve.” Mermel soon joined the company as president and chief product officer.
Meanwhile, the company settled on its core technology: a flexible implant with 1,024 electrodes that sits directly on the surface of the cortex. Called the Layer 7 Cortical Interface, it was designed to be implanted through a one-millimeter “micro-slit” cut surgically into the skull. Brain signals go to a decoder equipped with software “that’s sort of like Google Translate for brain activity,” Mermel says. The decoder listens in as the brain thinks about moving or speaking and then converts cognitive intentions into digital commands.
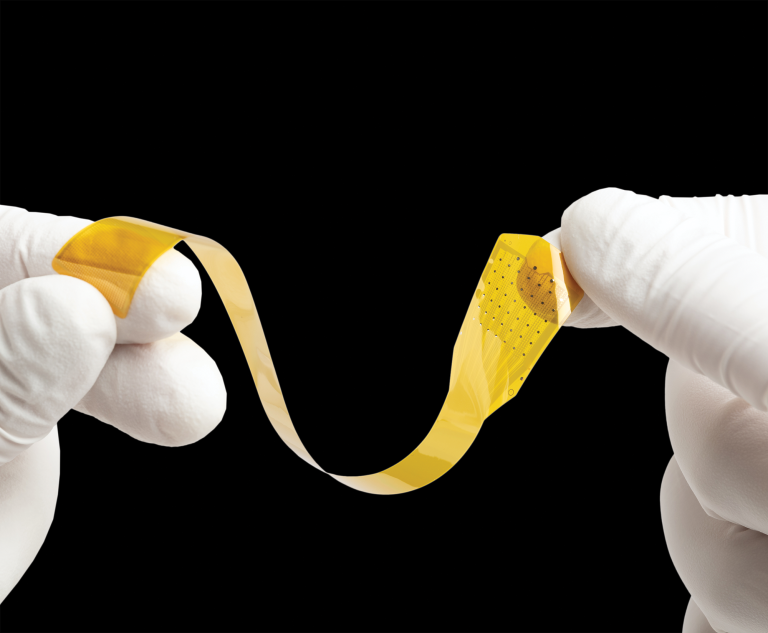
Practical technology
Precision Neuroscience launched officially in 2021 after raising $12 million in start-up funding. The company began testing in humans two years later — first at West Virginia University’s Rockefeller Neuroscience Institute and then at the Icahn School of Medicine at Mount Sinai and the University of Pennsylvania’s Perelman School of Medicine. During studies so far, Precision Neuroscience has teamed with neurosurgeons who agree to evaluate the technology while operating on patients for unrelated procedures. It ordinarily takes longer for BCI companies to enter the clinic. But the Precision implant is reversible — it can be removed without causing damage — and so the company faces a shorter path to regulatory clearance.
Iahn Cajigas, MD ’14, a neurosurgeon at Penn who has known Rapoport and Mermel since his own time as an HST student, has tested it in five patients so far. Surgical patients in his operating room engage with the technology while they’re awake. In one case, a man undergoing deep-brain stimulation for Parkinson’s disease was implanted with the device and then asked to perform hand exercises while wearing a sensor-equipped glove. Researchers working with Cajigas matched the patient’s brain signals to his movements, thereby “ground-truthing the data so that what we predict matches with reality,” Cajigas says. The implant is removed when the surgery is complete.
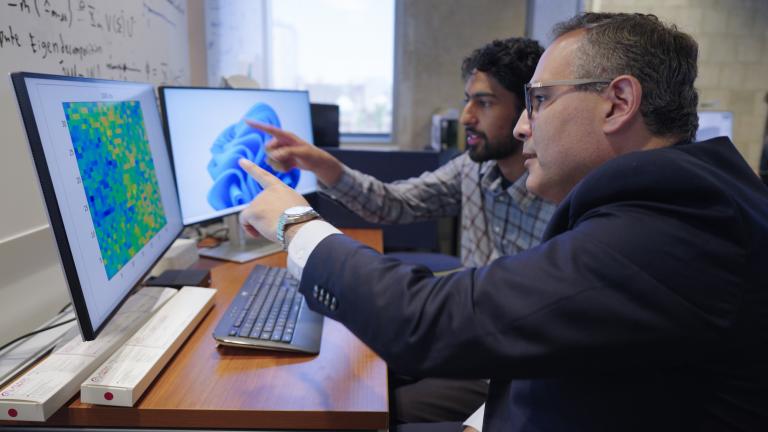
In the near term, Precision plans to bring to market a wired version of its device — one in which the cortical interface and back-end electronics are directly connected — as a diagnostic tool for neurological conditions, including epilepsy. The ability to record brain activity at high spatial and temporal resolution “will allow us to redefine our understanding of how the brain coordinates complex behaviors,” Rapoport says. Then, within the next two years, the company plans to submit a wireless system for regulatory approval, geared this time to patients with paralysis. The implant sits between the skull and scalp, where it amplifies and records neuronal signals. The signals are transmitted to software that controls a computer cursor or a prosthetic limb.
“Right now, if you’re paralyzed, it’s very difficult to operate a computer at the speeds that are necessary to hold a typical desk job,” Mermel says. “We want to restore a person’s ability to do that so they can be citizens of the digital world.”
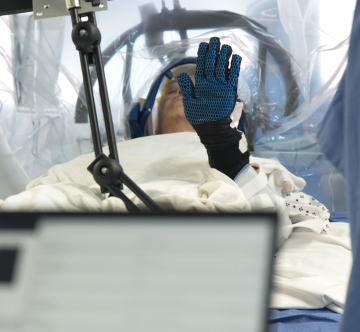
All implantable devices need to undergo cybersecurity risk assessments as part of the FDA-approval process. But Mermel says the company plans to go further by taking additional steps to ensure that neural data aren’t used in ways that haven’t been explicitly authorized by patients. “Core to this issue is building data security into the device from the ground up,” he says. “That being said, we are not reading the person’s inner thoughts in a way that they can’t control or wouldn’t have agency over.”
The BCI industry is rapidly growing, with investments predicted to increase from about $1.7 billion in 2022 to $6.2 billion by 2030, according to recent projections from the World Economic Forum. Research is focused in multiple domains, even gaming. Musk has famously stated that high-bandwidth connections to the brain may one day allow people to mesh with artificial intelligence.
Rapoport downplays these kinds of aspirations. “I don’t want to stray too much into the science fiction world of neural interfaces as elective procedures for able-bodied people,” he says. “What we’re working on is medical technology for the foreseeable future.”
Charles Schmidt is a writer based in Maine.
Images: Greg Dunn (Cortex in Red); Hannah Yoon (Rapoport); Penn Medicine (patient moving hand and Iahn Cajigas); Kevin Brown (Layer 7 Cortical Interface); Jay Watson (Mermel)