Dream Machines
Sometimes, answering the questions of science requires creating new ways of seeing
- 20 minute read
- Feature
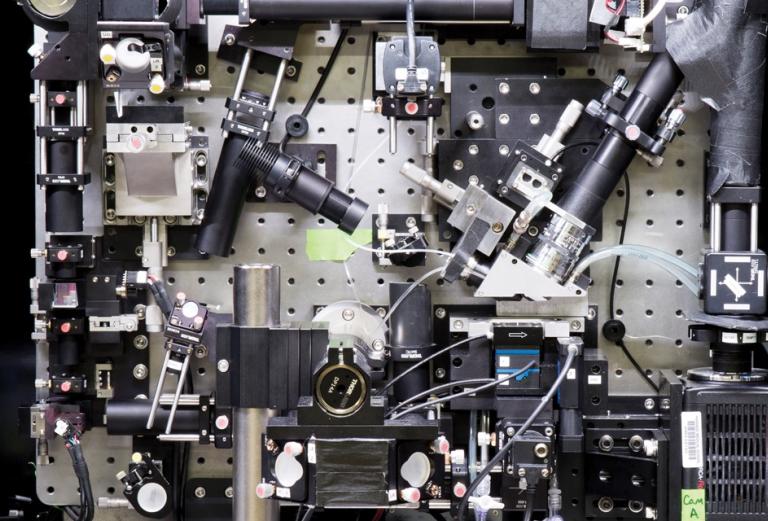
Bernardo Sabatini vowed that when he moved into his new office, he wouldn’t clutter it with microscope parts.
The resolution didn’t stick.
“This is a piece of a laser-scanning microscope I was building,” he says of a black contraption beside a family photo on his bookshelf. The guts of other instruments litter his desk and windowsill.
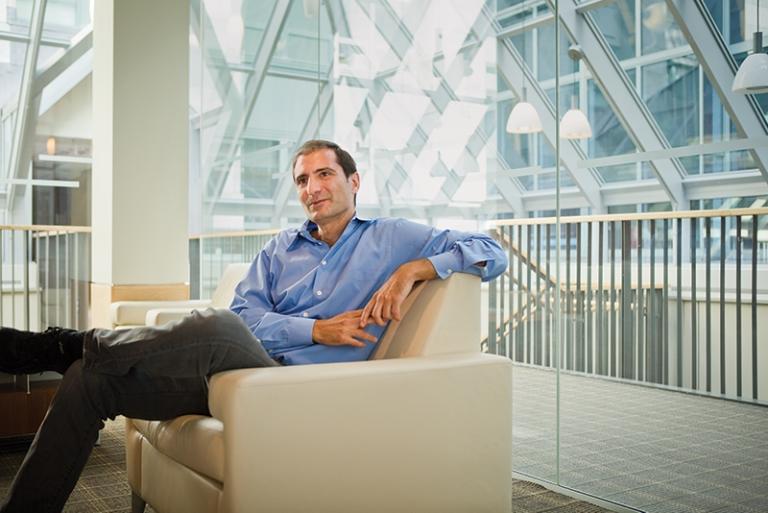
Originally a biomedical engineer, Sabatini, MD ’95, PhD ’99, the Alice and Rodman W. Moorhead III Professor of Neurobiology at HMS, builds imaging devices because it’s fun, because he hates having trainees expend their talent on labor-intensive tasks that more sophisticated equipment could do for them, and because he’s frustrated by questions about the brain he can’t answer with existing technologies. He’s currently trying to stitch together three microscopes to get a broader picture of synaptic circuits.
“A lot of our ability to get data that other people don’t have comes from our having built the hardware, designed the optics, and written the software,” he says.
“Plus,” he confesses, “I just love tinkering with stuff.”
Over in cell biology, Tomas Kirchhausen admits to similar tendencies.
“I’m a borrower,” says Kirchhausen, an HMS professor of cell biology and the Springer Family Chair of Pediatrics and senior investigator at Boston Children’s Hospital. His inclination to find and modify imaging instruments that can better serve his science has made him one of the people deeply involved in importing the latest visualization devices to the Quad.
Faculty and staff across HMS are adapting, combining, optimizing, and designing new visualization technologies to solve problems in biology and medicine. The fruits of their innovation range from microscopes to sample-preparation techniques to image processing and analysis.
Whether the components are tweaked or custom-made, excitement about the capabilities of modern optics is running high on campus as researchers and clinicians catch sight of phenomena they’ve been chasing for years, using the images and videos they capture to not only evaluate but also generate new hypotheses.
“Microscopes now can be used not just for passive observation but to induce changes in living tissue,” says Aurélien Bègue, co-director of the Neurobiology Imaging Facility and an instructor in neurobiology at HMS. “You become an active player.”
Imaging innovation convenes departments and institutions, which increasingly pool resources as devices require too much money or expertise to duplicate in every group. At the same time, many labs that innovate do so to bring down costs and provide the right balance of simplicity, flexibility, and effectiveness for users.
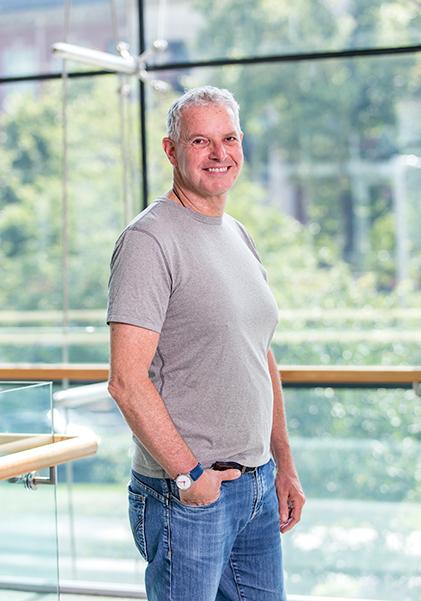
Innovation also arises from multidisciplinary collaborations and attracts people who blend traditional specialties. Such individuals tend to share a zeal for bricolage and for tackling problems, trying new things, remaining optimistic that solutions will eventually present themselves, and in the case of administrators, nurturing creativity in their labs.
The result: “We can look at life,” says Kirchhausen. “Today is like the Renaissance, when people were exploring the world. Genomics, proteomics, transcriptomics, these are snapshots of composition. We can now bring cell dynamics into that. And that’s exquisite. I wish I could live a hundred years longer.”
Fresh, not faded
Sandro Santagata, an HMS assistant professor of pathology at Brigham and Women’s Hospital, has been collaborating with HMS faculty to explore the possibilities of new imaging technologies for pathologists and compare the findings to hematoxylin and eosin (H&E) stains—the purple- and pink-hued tissue slides that have formed the backbone of clinical diagnosis for more than a century.
Nathalie Agar, an HMS associate professor of neurosurgery and associate professor of radiology at Brigham and Women’s, uses mass spectrometers to produce images that reveal incredible molecular heterogeneity within tumor samples. The first time Santagata saw one of Agar’s slides, he marveled at how quickly and clearly he could distinguish tumor from nontumor.
“The molecules have always been there, but we’ve never detected them in this fashion or used them for these applications,” he says. “You start to see the power of these techniques. There is a flood of molecular information, and we’re just at the beginning of knowing what to do with it.”
Meanwhile, Peter Sorger, the Otto Krayer Professor of Systems Pharmacology at HMS, wants to extract more information from cell cultures and patient tissue samples to better understand how different cells and individuals respond to drugs.
The typical method has been to use antibodies to attach fluorescent tags to molecules of interest. But since fluorophores usually come in only a few colors, ones that don’t interfere with each other, most of the time researchers can study only two or three proteins in a given sample. That’s no longer sufficient for those who seek a broader picture of cellular responses.
“To understand what’s going on, you need to expand your capacity. You try to measure multiple things at a time,” says Jia-Ren Lin, a research associate in therapeutic science in the Laboratory of Systems Pharmacology.
Lin relaxes by inventing things. He cobbled together a technique that allows researchers to apply fluorophores to three markers and snap microscopy images, then deactivate the tags in a chemical bath and apply three more—for up to twenty rounds and a total of sixty markers, depending on tissue fragility.
Software written and adapted by Sorger’s group combines the layers and allows scientists to manipulate 3D digital reconstructions of their samples, turn markers on and off, and otherwise explore terabytes of biological information distilled into vibrant images.
The team dubbed the method cyclic immunofluorescence, or CyCIF, and it has taken the HMS pathology community by storm since Sorger published the protocols, software, and initial results online for free in May 2018.
Commercial equivalents of CyCIF exist, but they’re expensive, can’t run as many cycles, and sometimes use proprietary antibodies, says Lin. By contrast, CyCIF can be performed with a few chemicals found at local pharmacies and hundreds of antibodies available on the market. It can be combined with whichever microscope a researcher has in her lab.
“Our having done this inhouse is not intended to prevent commercialization but to accelerate it,” says Sorger. “The hope is we’ll develop a clinically useful test so that when a patient comes in with brain cancer, somebody like Sandro can quickly generate a twenty-color image to diagnose them.”
Santagata believes Sorger’s and Agar’s innovations are ushering in a long-awaited era of quantitative pathology.
“We can be very precise now with measuring the amounts of markers, the numbers and densities of cells, and the relationships of cells to one another, which we were never able to do with immunohistochemistry,” he says.
This year, Santagata co-authored a case study with Sorger, Lin, and neuro-oncologist David Reardon at Dana-Farber Cancer Institute, in which they reported using CyCIF to measure immune cell distribution and signaling in a patient whose rare brain tumor kept returning despite multiple surgeries and experimental treatments. The images indicated a specific immunotherapy would work.
The patient is now in remission.
Basic science applications are equally promising. Sorger is eager to explore the relationships among tumor, microenvironment, and immune invasion, while Santagata thrills at the amount of tissue now available for analysis: CyCIF can be run on top of H&E, even on decades-old samples.
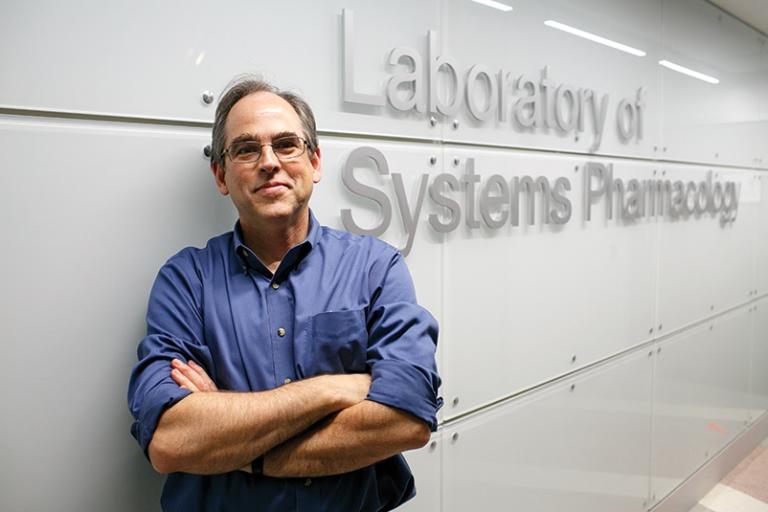
“Nobody had ever seen pictures at that resolution,” Sorger says of the first time they performed CyCIF on an archival breast biopsy. “Nobody even knew data existed at that resolution.”
Road trip
For decades, Kirchhausen had built world-leading expertise in the mechanics of how cells envelop incoming viruses, bacteria, and toxins in vesicles coated with the protein clathrin. He determined the atomic structure of clathrin. He peered at the process, called clathrin-mediated endocytosis, through the best microscopes available. He partnered with animators, sculptors, and musicians to creatively interpret the steps involved in its mechanism of action. He laid the foundation for a compound used today to block endocytosis. But even the most advanced technologies left a lot to the imagination.
Then Kirchhausen viewed his lifelong interest through a lattice light-sheet microscope.
“Oh, my God,” he recalls. “I could see all the little guys, the viruses, going in. I could see every single event in real time throughout the whole surface of a cell. Seeing that had been a dream of mine for I don’t know how many years.”
Light-sheet microscopes debuted in the 1990s as a solution to photobleaching in live cell samples. Other microscopes illuminate the entire sample while the lens captures one plane of tissue at a time; by the time a researcher reaches the last plane, the sample has lost quality from extended illumination. Light-sheet microscopes emit a microns-thin sheet of laser light that illuminates only the plane being imaged, keeping exposure times short and prolonging sample freshness as the tissue sweeps through the lens’ line of sight. The sweep repeats at intervals for hours or days. The planes are then computationally combined into a 3D movie that shows what’s happening in the cells over time.
“I think light-sheet is the most exciting thing going on in optical microscopy right now,” says Talley Lambert, a research associate in cell biology and manager of the Cell Biology Microscopy Facility at HMS. “Anybody doing live imaging of single cells or small chunks of cells has something to gain from this technology.”
Several iterations of light-sheet microscopes have appeared in recent years. The lattice light-sheet microscope was devised in 2014 by Howard Hughes Medical Institute (HHMI) investigator Eric Betzig, who won the Nobel Prize in Chemistry that year for co-inventing super-resolution microscopy. The lattice employs intricate math and physics to produce a thinner light sheet without shrinking the length of the plane, making it especially useful for subcellular imaging. Kirchhausen’s group built one at HHMI’s Janelia Research Campus under Betzig’s guidance and drove it to Boston. One year later, in 2015, six HMS basic science departments contributed to purchasing the parts for a second, which Lambert helped build.
Kirchhausen uses his lattice light-sheet to uncover details about endocytosis, viral entry, and cell division that have eluded observation until now.
“I thought I was an expert. Turns out, when we repeat an experiment using what we think are the same conditions—whoops, there’s something new, some surprise.”
Still, he remains thirsty for improvement. Earlier this year, Kirchhausen’s group worked with Betzig’s to enhance lattice light-sheet with adaptive optics, a technique developed by astronomers to sharpen images of the cosmos taken from Earth’s surface. In a telescope, a laser beam creates a false star—a control—so computers can correct for atmospheric distortions and make celestial objects appear more clearly. Similarly, a laser added to the lattice light-sheet allows researchers to “de-fuzz” their area of interest by compensating for the cellular material that lies between it and the lens.
“Now the revolution is complete,” says Kirchhausen. “We can not only see cells with close to single-molecule detail, but we can do the same in a cluster of cells. We can look at cells in their natural environment.”
Srigokul Upadhyayula, an HMS instructor in pediatrics working in the Kirchhausen lab, is building an adaptive optics microscope that is a complete redesign of the one at HHMI. It will combine the functionality of several instruments, including super-resolution and two-photon microscopy, to form a sort of Swiss Army knife mega-microscope that can capture an immense range of temporal and spatial resolutions during a single experiment.
Upadhyayula, who is also a member of a team overseen by Betzig, continually evolves Kirchhausen’s lattice light-sheet to make it more capable, compact, user-friendly, and economical without sacrificing quality. Always, the modifications address roadblocks that arise during research.
“I’m an engineer at heart. I always grow when I’m trying to solve a problem,” he says.
Light captivates Upadhyayula. He began his PhD studying solar energy and then turned to biological imaging, fascinated by modern microscopes’ ability to manipulate light. Now he helps his collaborators answer the question: When can you believe what you see?
Understanding how light interacts with each lens and mirror helps him build and calibrate today’s exceptionally complicated microscopes, which in turn gives him a deep appreciation for the instruments’ limitations.
“Operating a microscope is not just about knowing which button to push,” says Bègue of the Neurobiology Imaging core. “We’re getting to a point where the science we’re trying to do is not so simple. You need a good grasp of how these microscopes operate in order to do cutting-edge experiments. And you need to know your optics in order not to make mistakes: It is easy to treat an image the wrong way. In order to do good science, you need to apply the same rigor to microscopy and image processing that you do to statistical analysis.”
Swept away
There are alternatives to adaptive optics for handling soft-tissue distortion: You can also simply remove unneeded tissue.
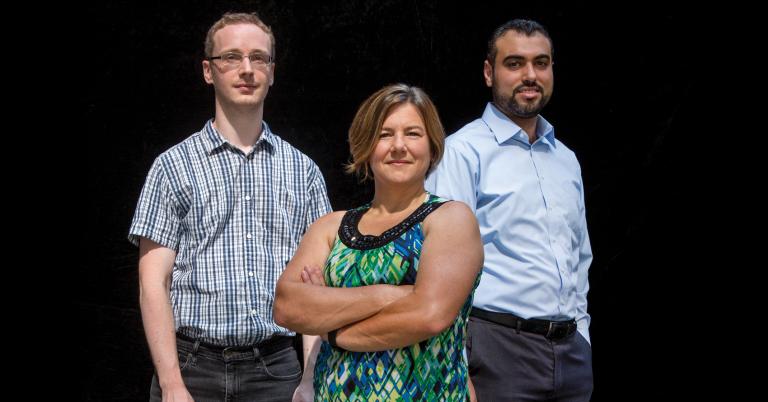
Consider the brain. Like a gelatin salad swirled with whipped cream and fruit, it’s filled with fats and other molecules that scatter light rays before they can reach a microscope lens, says Michelle Ocaña, co-director of the Neurobiology Imaging Facility and a senior imaging specialist at HMS.
A set of techniques known as tissue clearing wash away or bleach these inclusions and leave the natural protein scaffolding in place. The result: a whole, transparent organ with key structures intact. Researchers then add stains or use genetically expressed fluorescence to study structures of interest without anything in the way.
In late 2017, Ocaña’s group started offering tissue clearing to the Quad. Requests streamed in. So far, the team has helped researchers clear rat brains as well as mouse brains, jaws, prostates, kidneys, intestines, and spinal cords before imaging them with a simple light-sheet microscope.
“It’s booming. My feeling is, whatever you want to clear, let’s try,” says Mahmoud El-Rifai, a technician in the facility who specializes in tissue clearing and array tomography.
The biggest challenge yet arrived this summer when a postdoctoral fellow in the lab of Wade Regehr, an HMS professor of neurobiology, wanted to investigate whether the meninges enclosing a rodent brain contain axons and neurons. Previous attempts had failed because the delicate membranes tore whenever researchers removed the skull to observe them.
El-Rifai thought, why not try clearing the whole skull and brain together? That way “you don’t have to peel anything; everything remains intact,” he says. No one had done that before, but he thought it would be worth the attempt.
“The results were unbelievable,” he says. “Everything became so clear you couldn’t recognize it. We stained the brain and got some nice images, and the researchers saw what they needed to see.”
El-Rifai believes his inclination to embrace the unfamiliar and take risks arises from both the lab culture and his personal history.
When his family moved to the United States from Lebanon in 2010, El-Rifai was 18 years old and spoke no English. He started taking language classes, got a night job in a bakery, and put himself through college, graduating in four years with a degree in biomedical engineering.
“It was exhausting,” he admits, “but my thought was: work hard and you’ll find something at the end. You’ve got to keep trying, because nothing will work for you the first time.”
His philosophy applies to array tomography as well, the painstaking technique he swiftly mastered. Whereas tissue clearing works best on a large scale, array tomography, invented in 2007, provides high-resolution 3D images at the molecular level: “Where the secrets are,” says El-Rifai.
After a tissue sample is fixed and embedded in plastic, El-Rifai slices it into 70-nanometer sections, each attached to the next and lined up on a slide. It can take a year to section one axon. Imaging the sections one by one ensures that each receives the same amount of stain and light. Then the images are stacked via software, producing terabytes of data.
Not content with becoming an expert in new and difficult imaging technologies, El-Rifai alters them to meet people’s needs. He modified array tomography protocols to accommodate a zebrafish researcher’s hydrophobic resin and is helping another colleague experiment with staining RNAs instead of proteins. After discovering that many researchers choose one particular tissue-clearing technique because it has a lot of documentation about supported antibodies, he began compiling a similar database for a less popular but equally valuable technique. He hopes it will help people select the method that’s best suited to their projects.
A little to the left
It’s dark in the cell biology microscopy room. Fans from cooling units and computers provide a steady background hum as Lambert focuses on a sample—in this case, a fluorescent bead on a glass slide.
Lambert snaps a picture with the instrument, which resembles an elaborate erector set more than a traditional microscope. The image of the bead is a little blurry. He adjusts a knob and takes another photo: still not quite right. He tweaks a different knob: better, but room for improvement. He swaps out a lens and tries again. He’s so engrossed he doesn’t notice his colleagues coming and going.
Lambert doesn’t care about the bead itself. Rather, he’s intent on pushing the microscope to its full potential.
“I love tinkering on the microscopes,” he says. “I love making a more beautiful point spread function,” the image of an infinitely small dot. The cleaner he can make it, the better his microscope is performing.
“A lot of labs are microscope builders,” he says. “I am a microscope optimizer.
“People who design new ones, they drop it when they’re done and go on to the next one. I am more than happy to pick it up and evaluate whether it is truly useful. If it is, I will put a lot of time and effort into optimizing and democratizing that technology.”
As part of a core facility team assembled by Jennifer Waters, director of microscopy and an HMS lecturer on cell biology, Lambert has tackled recent microscopes including super-resolution, structured illumination, and several kinds of light-sheets. Yet, it’s just as important to him to improve upon existing technologies as it is to introduce new instruments to the Quad.
While some novelties, like light-sheet, fill a niche, he says, some older technologies remain underexploited, sometimes without researchers’ awareness. Colleagues have come to the core asking for super-resolution microscopy only to have Lambert discover that confocal could do the job with some fine-tuning.
“We need to better use what has been developed,” he says. “That’s sometimes not as exciting as designing a whole new technique, but it’s more far-reaching, and it’s far cheaper. Optimization definitely should come before innovation.”
Lambert began his career as a neurobiologist, studying learning and memory and synaptic morphology, until, as a postdoctoral fellow, he realized he enjoyed methodology more. His foundation in biology helps him meet collaborators in the middle, discussing their research goals in enough detail that he can advise on the best technology for the job.
In the neurobiology core, Bègue credits his own background in pharmacy and neuroscience, which he studied in addition to math and engineering, for allowing him to understand colleagues’ needs well enough to build solutions for them. He derives similar satisfaction from helping.
“One thing I regret as a biologist is that I don’t have a lot of questions that keep me up at night,” he says. “But when I meet other scientists and I can say, ‘We’re going to find a way to help you with that question that has been haunting you for the past ten years,’ that’s very satisfying to me.”
Even as they recognize the value in centralizing expertise, Bègue and Lambert share a passion for making imaging technology more accessible. Lambert writes software, designs graphical user interfaces, authors review articles, simplifies tools, and lowers costs, while Bègue plans to organize basic optics seminars and host in-depth consultations with Quad labs to discuss their imaging challenges.
Tinkering with microscopes provides plenty of experience in overcoming such challenges.
“Problem solving is great, but it means you’re always dealing with a problem,” says Bègue, who builds and modifies devices for himself and others. “You will try many solutions and 99.999 percent of them will fail. Not everyone is built to handle that much frustration.”
Rather than throwing his equipment, or himself, against the wall, Bègue starts by throwing every idea he can think of at a problem, tries to learn from each failure, and waits for small successes he can build upon. He also breaks down complex challenges into smaller components.
“Someone will say, ‘We want a microscope that will do x, y, and z, and make coffee too,’ ” he quips. “I start by trying to do x. If that works, I’ll see if I can add y to it.”
Bègue draws confidence from the fundamental laws governing optics. “The good thing is I have physics on my side,” he says. “We have centuries of knowledge about light. You know it’s eventually going to work because physics says it will.”
The downside is that if nothing works, he knows he’s the one to blame. “With biology, you can say, oh, maybe my mouse behaved differently today. Well, light does not decide to do something different.”
Lambert, too, knows the pain of beating at a problem for days and then, “if you’re lucky, you have a microcosm of a eureka moment that improves that teeny process.” The joy lies in “figuring stuff out.”
Best laid plans
Like many neurobiologists, Sabatini wants to move from studying single neurons or small clusters to observing thousands of neurons at once, catching in action the circuits that underlie behavior.
He has already advanced the field through innovations in optogenetics, a technology that activates desired neurons with light, and two-photon microscopy, a high-resolution 3D fluorescence imaging technique. Earlier this year, his lab created peptides that photoactivate so researchers can study the function of natural opioids produced by the brain. But there’s a long way to go.
Right now, he says, he’s completing “a beast of an instrument” that should help with several burning questions. The first part contains the same beam-emitting device found in lattice light-sheet microscopes, which Sabatini uses to swiftly map all the neurons in a tissue sample. The second part is a holographic optogenetic stimulation microscope, which lets Sabatini create 3D patterns of light that activate whichever presynaptic neurons he wants. He can then record the downstream effects in postsynaptic neurons using the third component, invented by an MIT colleague: a two-photon microscope combined with an electrode-implanting robot.
The as-yet-unnamed behemoth is limited to five or ten electrodes at a time, but from those, Sabatini expects to be able to infer the activity of hundreds to thousands of neurons per experiment. He’s itching to illuminate a “really cool” type of neuron his lab discovered that releases multiple, seemingly contradictory, neurotransmitters; other instruments haven’t found the chemicals’ targets nor revealed why they simultaneously excite and inhibit. He’s also keen to investigate whether another class of neurons changes the ratio of neurotransmitters released when an animal experiences something new, teaching it to seek similarly good, or avoid similarly bad, experiences in the future.
When those projects get underway, he plans to build a second version of the microscope to help a cross-institutional team at HMS, the Broad Institute of MIT and Harvard, and the Harvard Stem Cell Institute analyze how genetic variation changes functional neurocircuitry in brain organoids. Additional colleagues wait in the wings.
“There are a lot of problems waiting for this machine,” says Sabatini.
He just needs to get the pesky optics aligned first.
Then it will be on to troubleshooting software issues—and preparing to create whatever instrument their research demands next.
Stephanie Dutchen is a science writer in the HMS Office of Communications and External Relations.
Images: John Soares