Inflammation and Brain Health
Normally a guard against brain injury and infection, inflammation is increasingly being implicated in cognitive decline
- 11 minute read
- Feature
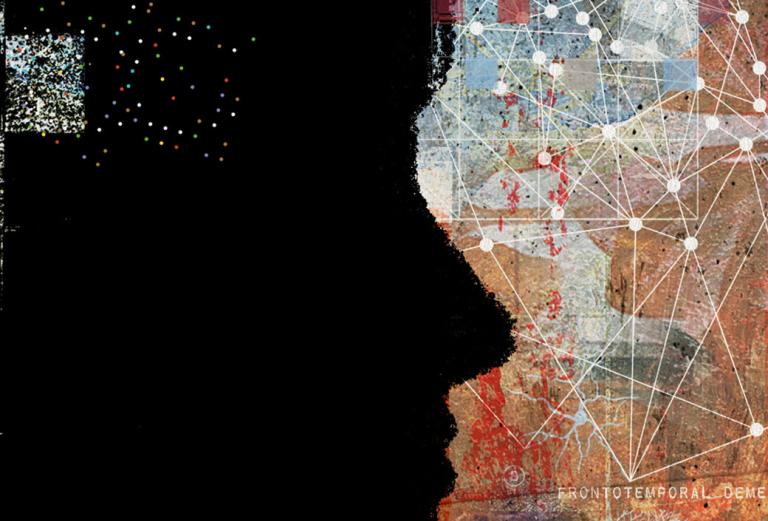
During his internship at Beth Israel Hospital in the mid-1970s, Eric Larson, MD ’73, recalls that it wasn’t unusual to say that older people with cognitive decline had senile dementia or hardening of the arteries.
These catch-all phrases are rarely used today, however, as evidence increasingly connects age-related cognitive symptoms to neurodegenerative changes in the brain. Larson has watched, and been part of, this evolution in thinking. Currently a senior investigator at Kaiser Permanente’s Washington Health Research Institute in Seattle, Larson founded Adult Changes in Thought, a prospective cohort study that tracks brain aging and dementia in approximately 5,700 participants from the local population.
A striking observation from the study, he says, is that some people remain cognitively healthy despite what appear to be debilitating neuropathologic changes seen at autopsy. One recent study participant in particular, a woman who enrolled in the study decades ago, was “still very on top of things and very with it” right up to her death in her mid-90s, Larson says. Yet, the autopsy of her brain showed a pathology that normally would have been diagnosed as Alzheimer’s disease. “How was she seemingly resilient given her brain’s degeneration?” Larson asks.
Findings like this, say scientists such as Rudolph Tanzi, PhD ’90, the Joseph P. and Rose F. Kennedy Professor of Child Neurology and Mental Retardation at HMS and Massachusetts General Hospital, underscore the powerful role of neuroinflammation in conditions such as Alzheimer’s disease, Parkinson’s disease, Lewy body dementia, and frontotemporal dementia. Rates for these neurodegenerative illnesses are increasing steadily with the aging of the U.S. population, and it is thought that worsening symptoms can be blamed on the havoc inflammation wreaks on brain regions involved in thinking, memory, and movement, or, in the case of frontotemporal dementia, personality, behavior, and language.
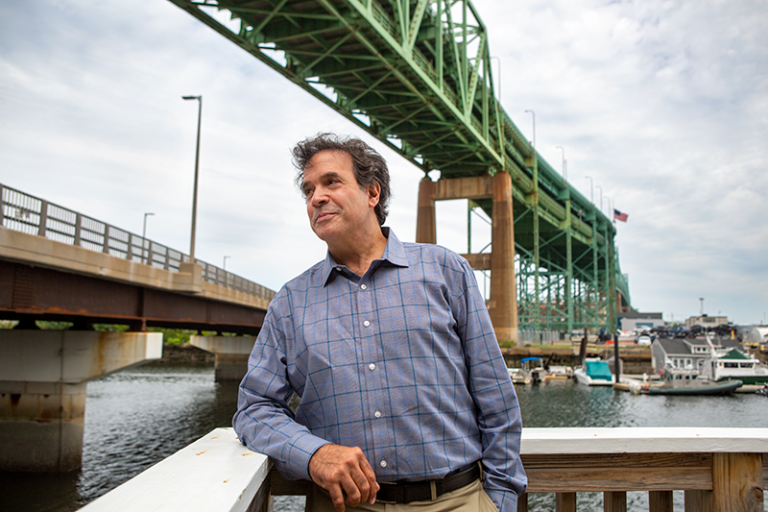
Harmony lost
Coordinated in part by the brain’s resident immune cells—microglia and astrocytes—neuroinflammation ordinarily protects against infection and injury. But when spinning out of control, it “can kill ten times more neurons than the pathological changes that set it off,” says Tanzi, who also co-directs the McCance Center for Brain Health at Mass General. Scientists have linked neuroinflammation with cognitive decline and higher risks for age-related cognitive impairment. And autopsies show clear evidence of it in the brains of people who have died from Parkinson’s disease. Conversely, signs of neuroinflammation in the brains of people who do not exhibit Alzheimer’s symptoms “are for the most part nonexistent,” says Tanzi, despite their having other disease-related pathologies.
Beth Stevens, an HMS associate professor of neurology, a research associate in the F.M. Kirby Neurobiology Center at Boston Children’s Hospital, and a member of the Broad Institute of MIT and Harvard, says that neuroimmune signaling and its role in age-related neurodegeneration is galvanizing the field. “It’s a really exciting time to be studying this,” she says. “Immune pathways are clearly part of the story. And we need to figure out how and why.”
Much of the evidence so far has come from studies of Alzheimer’s disease, which, say Tanzi and others, dwarfs the other neurodegenerative conditions in terms of funding and research. The onset of Alzheimer’s is triggered by two proteins: amyloid beta, or A-beta, and tau. The precursor of A-beta ordinarily participates in neural growth and repair, while tau normally helps to stabilize the structure, or “scaffolding,” of neurons. Both proteins can become troublesome with age, however. Instead of dissolving away in solution, A-beta can start clumping together to form insoluble amyloid plaques between nerve cells. Similarly, tau can aggregate into structures called neurofibrillary tangles that also block nerve cells from communicating. In Parkinson’s disease, Lewy bodies, which are tiny round structures made of the protein alpha-synuclein, accumulate in neurons. And like plaques and tangles, the Lewy bodies are neurotoxic and therefore capable of triggering neuronal cell death, leading to an immune reaction.
Domino effects
Immune cells in the brain usually play housekeeping roles: Microglia consume debris, such as amyloid, and dead or infected cells and prune excess neuron-to-neuron junctions, known as synapses, allowing brain circuits to run smoothly. Astrocytes help build up the blood-brain barrier, which protects against infection, while also supplying neurons with nutrients. But like Dr. Jekyll and Mr. Hyde, microglia and astrocytes can take on more sinister personalities, during which they “throw off the apron, put on SWAT gear, and become killers,” Tanzi says.
These cells didn’t get the memo about how long we’re living now and that neurons die for reasons other than infection.
Francisco Quintana, an HMS professor of neurology at Brigham and Women’s Hospital, has spent years investigating what drives immune cells to flip like this. Quintana grew up in Argentina, and says he was inspired to get into neurology after watching his grandfather—whom he describes as a towering figure and a personal hero—succumb to a neurodegenerative disease.
Quintana made an important discovery that describes how the inflammatory cascade can begin and perpetuate in the brain: pathological subsets of microglia and astrocytes communicate with each other to coordinate neuronal attacks. In some cases, these attacks can be instigated by T cells, a type of white blood cell important in the immune response, entering from outside the brain. Quintana found that when T cells secrete a specific protein, that protein acts to shift transcriptional profiles in astrocytes, causing them to turn on genes that trigger inflammation. When activated in this way, astrocytes spew out free radicals, such as nitric oxide, which in turn activate microglia, making them become neurotoxic as well. By engaging in this cross talk, astrocytes and microglia launch a chronic assault on brain tissue that persists even without further T cell involvement. The inflammatory reaction, Quintana says, “takes on a life of its own.”
Tanzi’s research shows that neuroinflammatory cascades can also occur in the opposite direction, with microglia activating astrocytes, which in turn attract T cells. In 2008, Tanzi identified one particular gene, called CD33, that serves as an on switch for Alzheimer’s disease-associated neuroinflammation. Located on microglial cell surfaces, “this is the ‘bad-guy’ gene that tells the cell to produce a bunch of cytokines to get astrocytes involved in the killing,” he says. Cytokines are proteins that mediate the body’s immune reaction, usually by modulating it, but, at other times, by increasing it.
In later research, Tanzi showed that CD33’s activity coordinated with TREM2, a complementary “good-guy” gene in microglia. If the expression of TREM2 increases, the microglial cells remain as housekeepers. But if TREM2 is mutated, or otherwise defective, CD33 can switch the microglial cell to an inflammatory mode. Mutations in TREM2 have been linked to Alzheimer’s disease and to Parkinson’s disease.
Inside and out
Why would the brain’s immune cells become so destructive in the first place? One explanation is that they haven’t yet adapted to increasing human life spans. The cells were programmed during evolution to eliminate infections. So, when they see a dying neuron, Tanzi says, they simply assume it’s infected, and then shift from housekeeping to inflammation. “By wiping out affected parts of the brain, they’re aiming to keep the infection from spreading,” Tanzi says. “These cells didn’t get the memo about how long we’re living now and that neurons die for reasons other than infection.”
Importantly, different triggers can set these neuroinflammatory cascades in motion; not just infection, plaques, tangles, and Lewy bodies, but also pollutants and physical trauma. Tanzi wears many hats, one of them being a brain health advisor to the New England Patriots football team. Among the responsibilities that come with that role, he says, is “trying to help these guys avoid later problems with chronic traumatic encephalopathy,” a neurodegenerative condition caused by repeated blows to the head. Like frontotemporal dementia, chronic traumatic encephalopathy is a “tauopathy;” it’s characterized by neurofibrillary tangles that form in the absence of amyloid plaques.
Pivot
Research connecting inflammation with neurodegeneration is still in its early days, and Bruce Yankner, an HMS professor of genetics and neurology and co-director of the Paul F. Glenn Center for the Biology of Aging, cautions that questions remain about the degree to which inflammatory processes can be lumped together in different conditions. “These diseases likely share common features, such as activation and proinflammatory responses of microglia and other cell types,” Yankner says. “But the way in which these responses overlap between diseases is very poorly understood.”
Also not well understood is how the brain protects itself from inflammation and age-related changes. Still, researchers are making headway. Yankner and his team published a study in 2014 that revealed one intriguing mechanism. They found it while investigating changes in age-related gene expression in the prefrontal cortex, the part of the brain responsible for executive functions such as planning and social behavior. Their research showed that a protein called REST affords some resilience against cognitive declines.
Yankner, who also is a professor of neurology at Boston Children’s Hospital, is well-known for his 1990 discovery that A-beta is toxic to neurons, a finding that led to what’s widely known today as the amyloid hypothesis in Alzheimer’s disease. According to that hypothesis, accumulating A-beta in the brain is the fundamental cause of Alzheimer’s and the driving force behind its initiation. With this newer finding, Yankner’s research team reported that REST is lost in patients with Alzheimer’s, but present in the brains of people who retain high cognitive performance until death, even if they have evidence of disease pathology at autopsy.
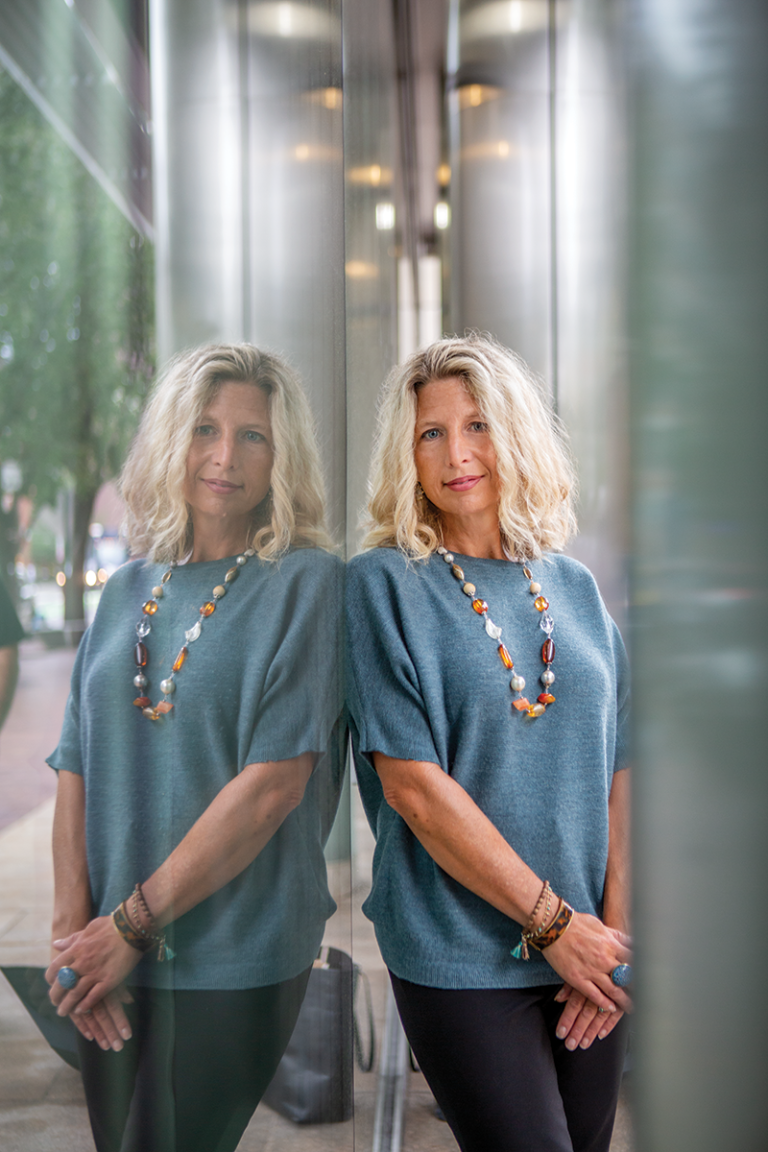
Findings like these galvanized researchers in the field, who are now “pivoting away from pathological hallmarks of disease toward neuroimmune pathways that are clearly part of the story,” Stevens says. In July, Tanzi and his colleagues published findings in Nature showing that a small subset of astrocytes can actually push back on the brain’s inflammatory responses and try to quell them. Making up about 4 percent of the total astrocyte pool, the cells in this specialized subset produce a molecule called interleukin-3 that binds to microglial cells and turns them back into housekeepers capable of removing debris such as amyloid. A crucial player in that helpful interaction turned out to be TREM2. According to Tanzi, microglial cells will go back to housekeeping if they detect interleukin-3 but that doing so requires them to express the molecule’s receptor.
“What we found is that TREM2 tells microglial cells to turn up the interleukin-3 receptor, so they can go back to being beneficial,” Tanzi says. Interleukin-3, he added, “has the potential to be a new treatment if we can figure out how to use it.”
In Stevens’ view, therapeutic opportunities like these will depend on a better understanding of how the brain’s immune cells shift transcriptional states to carry out particular functions. The cells have many different roles, she says, and will adopt varied states accordingly. “At the moment, we don’t know enough about whether it’s one population of cells that is detrimental, and if we should target those cells, or whether we should target their state, and shift them back to a more beneficial state,” Stevens says. “If so, we could target the bad and promote the good. And to get there, we need to isolate the specific states or populations of cells and understand their functions. That’s what the field is working on now.”
Ways and means
New technologies are aiding in that endeavor. For instance, scientists in the field are turning to single-cell RNA sequencing to track disease-associated transcriptional states in microglia and other immune cell types. Quintana used these tools to identify which subsets of microglia and astrocytes promote neuropathology, and he also relied on a technology called RABID-seq to study how the cells communicate with each other. Further opportunities come from culturing organoids, which are three-dimensional bits of brain tissue, in a dish. By using culture media containing the proper chemical factors, adult human cells—including those taken from patients—can be brought back to a near-embryonic state, then prodded to grow into any sort of cell from the body. Tanzi adopted this approach to create tissue models of Alzheimer’s disease, which he uses in a number of studies, including ones investigating how T cells in nerve tissue throughout the body infiltrate the brain and worsen inflammation.
Incorporating new neuroimmune biomarkers into ongoing clinical trials could be an exciting new direction and advance for the field.
His insights add to a growing body of evidence that neuroimmune signaling can reach well beyond the brain, even to the gut microbiome. While the vast assortment of bacteria, viruses, and other microbes inhabiting the digestive tract have many beneficial roles, “it can also amplify inflammation in the brain and make it worse,” says Howard Weiner, the Robert L. Kroc Professor of Neurology at HMS and Brigham and Women’s. In his book The Brain Under Siege, Weiner presents supporting evidence obtained from mice bred to mimic features of Alzheimer’s disease. Treating the animals with antibiotics altered their microglia, converting the destructive cell type to the protective type. Similarly, evidence suggests that Parkinson’s disease is related somehow to microbial changes in the gut, likely through inflammatory pathways. Weiner cites research using mouse models of Parkinson’s disease. When bred in germ-free conditions, the animals—which have virtually no gut bacteria—have fewer Lewy bodies in the brain and never develop problems with movement, one of the symptoms of Lewy body dementia. Weiner speculates this could be because microbially-induced inflammation somehow boosts the gut’s production of alpha-synuclein protein, which is then transferred to the brain via the vagus nerve. Adding to evidence connecting Parkinson’s disease to the gut-brain axis, Weiner writes, is that people with inflammatory bowel disease are also at higher risk of Parkinson’s.
Stevens asserts that further progress toward understanding the connection between inflammation and age-related neurodegeneration will come from using molecular biomarkers measured in the body’s fluids. She and her collaborators are analyzing blood and cerebrospinal fluid from people with neurodegenerative conditions and from those without such conditions, and are performing deep, large-scale comparative analyses of their proteins. Soluble forms of TREM2 can be detected in cerebrospinal fluid, “and there’s evidence that it changes in people with Alzheimer’s disease,” she says. “That’s an excellent example of a biomarker that’s being read out in cerebrospinal fluid now, and there are going to be many others as well. Incorporating new neuroimmune biomarkers into ongoing clinical trials could be an exciting new direction and advance for the field.”
Meanwhile, in Seattle, Larson and his colleagues are looking forward to the next phase of the Adult Changes in Thought study, which recently won a five-year federal grant totaling $55.6 million—triple its prior funding. Participants agree to baseline tests and to evaluations every two years. Many consent to donate their brains to research when they die. Larson points out that the study, which began in the late 1980s, is among the few studies that collect control brains from people who don’t develop dementia during study observation.
“The funding environment has dramatically improved and so has the technology,” he says. “We’ve learned a lot already, and this new support means that in the next five to ten years, we’re going to learn a lot more.”
Charles Schmidt is a writer based in Maine.
Images: Dung Hoang (top); John Soares