Looking into the Matter
Diagnosing brain injuries poses challenges that researchers are rising to meet
- 8 minute read
- Feature
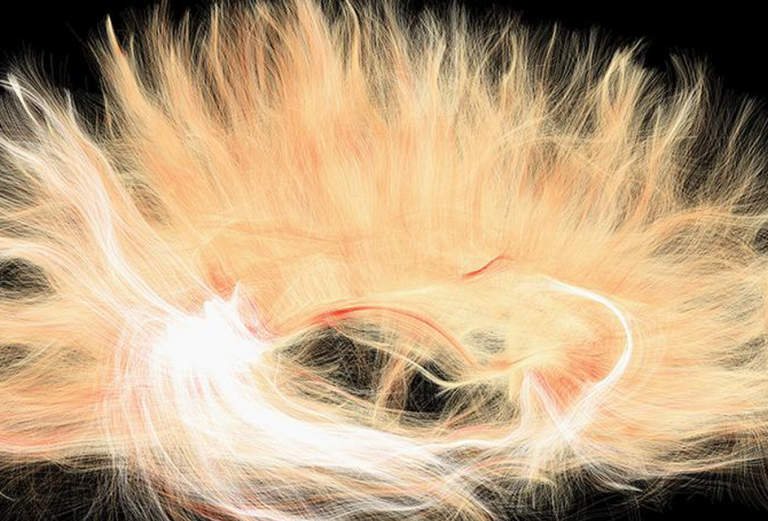
A version of diffusion tensor imaging was used to determine the pathways of white matter fibers that travel through the brain's corpus callosum.
Less than a handful of years ago, Joseph Giacino was readying for an appearance on the Charlie Rose program. The PBS show, which features discussions between journalist Rose and various newsmakers, had invited Giacino, director of rehabilitation neuropsychology at Spaulding Rehabilitation Hospital, and other experts to talk about the state of treatment for traumatic brain injury.
For about a decade, Giacino had been investigating this type of injury with the goal of discovering a way to help patients recover from its more severe forms. He had been exhaustively characterizing the capabilities of patients in minimally conscious and vegetative states and also had been searching for drugs that might promote recovery.
So when the show’s producers invited Giacino to talk about the state of the science in this field, they were confident they had the right expert. They may not, however, have been fully prepared for Giacino’s opening statement: “There’s not a single proven treatment for traumatic brain injury at present.”
Something from Nothing
Interest in gauging the damage from and devising treatments for traumatic brain injury has only increased since Giacino and Rose talked. Awareness of the toll that brain trauma takes among athletes and those in the military has crescendoed. In the past 12 years, in fact, an estimated 320,000 military personnel have experienced some form of neurological trauma during their deployments in Iraq and Afghanistan.
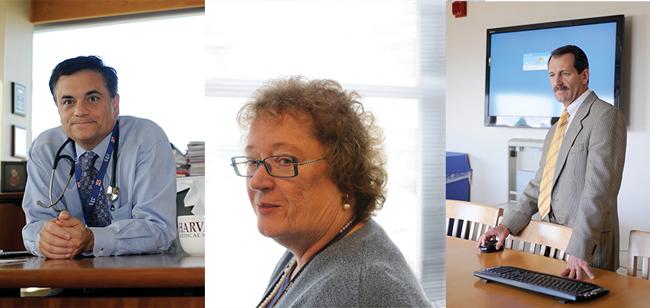
But researchers may be poised to provide physicians with ways to recognize even the mildest form of these injuries. New diagnostic tools in imaging, brain stimulation, and molecular biology are emerging, and, together with other technologies, are also giving researchers insights into how to develop targeted therapies.
Map Quest
These developments are welcome news to the medical community. Each year in the United States, 1.7 million people seek medical attention for traumatic brain injury. As large as that number may seem, it’s probably conservative because many people never seek help for concussions or similar mild brain injuries they incur. This knowledge leads many experts to call traumatic brain injury the “silent epidemic.”
Between 15 and 30 percent of those who suffer mild traumatic brain injury endure chronic headaches, depression, post-traumatic stress, and memory and cognitive impairments. Ross Zafonte, the Earle P. and Ida S. Charlton Professor of Physical Medicine and Rehabilitation at Spaulding Rehabilitation Hospital, likens these mild forms of brain injury to a disease: A light bump or the reverberations from a blast can trigger a chain reaction of inflammation and neurodegeneration that may continue for years.
Zafonte and Martha Shenton, an HMS professor of psychiatry and radiology at Brigham and Women’s Hospital who uses diffusion tensor imaging to study neurological disease, have been working to develop imaging tools that can detect these mild forms of brain trauma. When they began their collaboration in 2007, “there was nothing to see,” says Zafonte. Conventional imaging techniques, such as MRI and CT scans, did not show abnormalities associated with mild trauma.
The price of such blindness is high. X-rays help doctors diagnose fractures. Biopsies are used to diagnose cancer. “In medicine,” says Shenton, “you have to be able to detect and diagnose something before you can treat it.” Shenton and Zafonte hope diffusion tensor imaging will allow such insight. One of its measures, known as fractional anisotropy, works by measuring the rate and direction in which water diffuses in the brain.
Shenton demonstrates the concept by comparing the way a water droplet spreads on a tissue versus its movement on newsprint. On a tissue, water is not constrained; it spreads in all directions equally to form a perfect circle. On newsprint, however, water is constrained, moving along the fibers in the paper to form an ellipsoid. In the brain, water that is constrained has been stopped by a barrier, a fact that provides information about the structure of the tissue. Water is constrained along a fiber tract in white matter, for example, while cerebrospinal fluid provides no such constraints. The technology has proven useful for capturing images of some previously invisible neurological wounds. One such wound is diffuse axonal injury.
In diffuse axonal injury, the axon that extends from the body of a nerve cell tears or stretches, causing chemical imbalances and cytoskeletal disruptions that change the way water is constrained. These subtle changes can be captured by diffusion tensor imaging. Shenton’s lab has recently refined the technology, adding new analytical techniques that can distinguish neuroinflammation from neurodegeneration.
The information conveyed in a diffusion tensor scan also is more nuanced than that displayed by imaging tools such as x-ray. Unlike most skeletal injuries, brain injuries are highly variable. So Shenton and her team are building a brain atlas composed of averaged measures from scans of normal, uninjured brains. When a scan of a traumatized brain is compared to measures in the atlas, the measures of injured areas fall outside the norm.
In analyzing the scans, researchers are starting to see patterns emerge. “As we get more refined imaging techniques, we’re beginning to see differences between blast-related injuries, auto accidents, and falls,” says Zafonte.
Shenton and Zafonte are using the atlas in a randomized clinical trial to study whether the anti-inflammatory diabetes drug glyburide might be effective in reducing the neuroinflammation that results from mild traumatic brain injury. Shenton believes that soon the atlas could be used in the clinic to identify and diagnose brain injury.
Chip Ahoy
For Kit Parker, a founding core-faculty member of the Wyss Institute for Biologically Inspired Engineering and Harvard University’s Tarr Family Professor of Bioengineering and Applied Physics, an interest in understanding brain injury sprang from his military service in Afghanistan. “People were trying to kill me with IEDs, so I figured I better start working to understand the injuries those devices were causing,” he says.
Parker began researching the literature on integrins, a type of protein that anchors cells to their surroundings. Integrins are essential for the growth of cardiac tissues, Parker’s area of expertise; since coming to Harvard, he’s been working on heart-on-a-chip technology. These proteins translate mechanical signals from outside a cell into chemical responses inside a cell, a transformative ability that Parker thought might help explain the chemical cascade caused by a blast-induced brain injury.
His literature search yielded nothing. So he and a student sat down for coffee, and, hunched over a napkin, began sketching out what would become the first study of the role of integrins in brain injury. Their plan? Construct a brain-on-a-chip.
For their investigation, the researchers secured individual rat neurons to stretchy silicon and, in an effort to simulate the effects of a blast, subjected the silicon sheet to a quick pulse of energy. Parker found that forces too small to disrupt the cell membrane could still disrupt the focal adhesion complex—that is, the connections that integrins build from the cytoskeleton through the cell membrane and into the extracellular environment. Such a disruption triggers an injury-inducing signaling cascade within a neuron. Parker thinks that blocking this cascade with a neuroprotective agent of some sort would be an effective way to prevent progressive brain injury.
In Parker’s view, the search for neuroprotective interventions is crucial: improvised explosive devices aren’t going away anytime soon. On the battlefield, the need for medical tools that can be used to diagnose and mediate the injuries IEDs produce is devastatingly clear. “If I pull you out of a blown-up vehicle and you’re bleeding, I can use a tourniquet to help stop the blood loss,” says Parker. “But if you’re experiencing the earliest triggers of the chemical cascades that cause brain injury, I’ve got no first aid for that.”
Light Work
Michael Whalen, an HMS associate professor of pediatrics at Massachusetts General Hospital, is working on near-infrared brain stimulation that could be just the kind of first aid for brain injury that Parker is seeking. Whalen’s vision, however, centers not on a neuroprotective agent but on a device; specifically, a helmet that shines healing light on an injured brain.
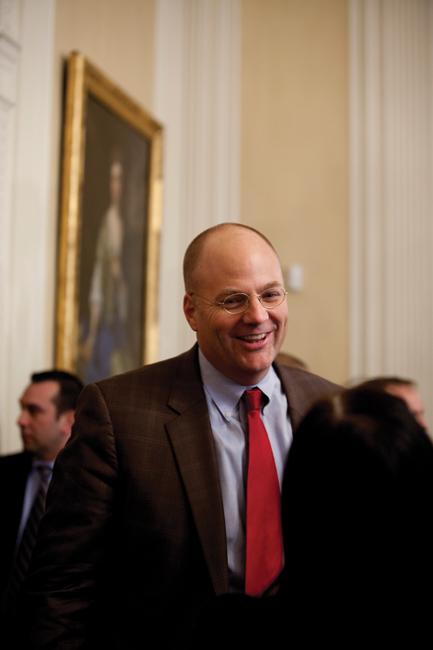
Whalen’s idea is grounded in work done four decades ago. Shortly after the first working laser was built, Hungarian physician Endre Mester used an animal model to determine whether skin, shaved of hair, developed cancer when exposed to laser light. To his surprise, he found that the hair on animals treated with the laser grew back faster than it did in a control group. Mester had discovered the phenomenon now called laser biostimulation.
Whalen uses the same principle to treat traumatic brain injury. By directing specific wavelengths of near-infrared light onto the heads of animals that experienced controlled cortical impact, Whalen has been able to improve the animals’ cognitive function outcomes and to reduce post-trauma brain inflammation.
Even though the therapy is considered a safe treatment for certain conditions in humans, Whalen continues to focus on perfecting the technique in animal models to better understand how cellular changes translate into neuroprotection and improved healing of brain injuries. If all proceeds as he hopes, Whalen expects to see neuroprotective helmets in the backpacks of army medics and on the sidelines of professional football games within the decade.
Fast Forward
Whalen’s research, together with the efforts of others in the field, have awakened interest in traumatic brain injury research. In May, Giacino, whose statement to Charlie Rose’s audience starkly described a field in need of progress, was part of a research team that produced the first successful results of a clinical trial of a treatment for this injury. The study, codirected by Giacino and John Whyte of the Moss Rehabilitation Research Institute, showed that the drug amantadine, now used for Parkinson’s disease, helps patients with severe traumatic brain injury recover more quickly. “We finally have evidence that we may be able to do something to help these patients get better,” says Giacino.
With new diagnostic and investigative tools, clinicians and researchers no longer need to reason around an impenetrable black box. As a result, the conversations in the field today are focusing on how to bring it all together: how to leverage new imaging tools and biomarkers to track patient progress in clinical trials, how to use brain-on-a-chip models to screen potential drugs, how to combine pharmaceutical and brain stimulation therapies, and how to know when to apply one or the other. For those with traumatic brain injuries, it all adds up to one healthy dose of hope.