The Long Journey of Gordon Freeman and Arlene Sharpe
The two immunologists have shared a lifetime of scientific exploration and discovery
- 13 min read
- Feature
Photo illustration: Dana Smith; photos: Jason Grow and courtesy of Arlene Sharpe and Gordon Freeman
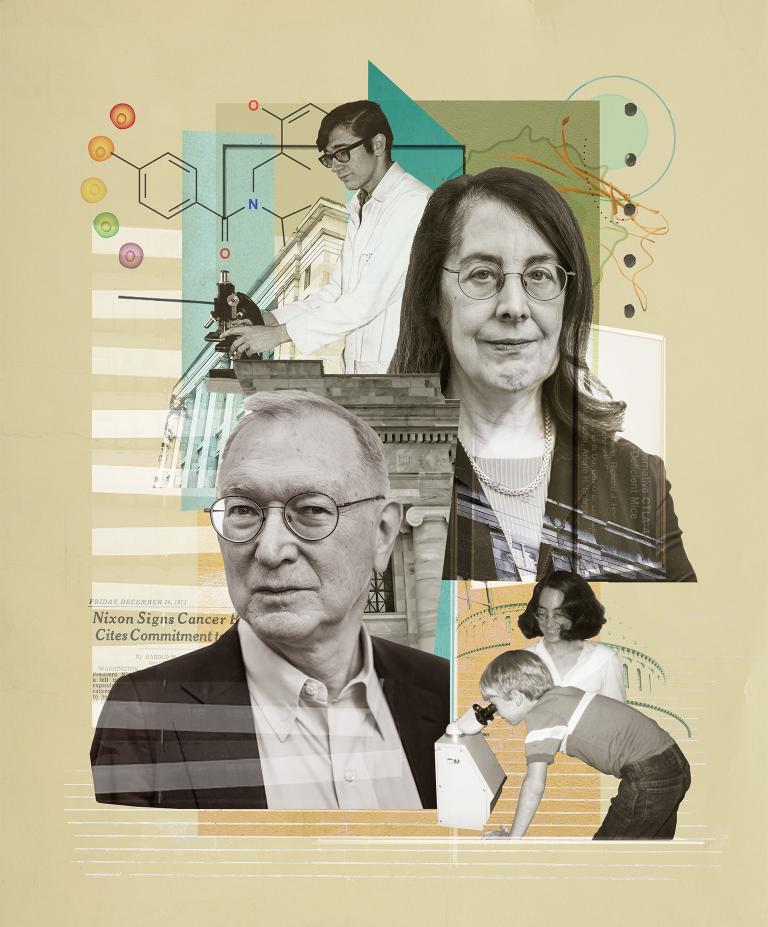
Photo illustration: Dana Smith; photos: Jason Grow and courtesy of Arlene Sharpe and Gordon Freeman
Work described in this story was made possible in part by federal funding supported by taxpayers. At Harvard Medical School, the future of efforts like this — done in service to humanity — now hangs in the balance due to the government’s decision to terminate large numbers of federally funded grants and contracts across Harvard University.
Picture two scientists, giants in their field, traipsing around the United Kingdom on a scenic adventure.
First, they stop at Stonehenge on the summer solstice and marvel with the thousands of others gathered to greet the rising sun. Next, they wander through the rolling hills, browse the charming shops, and admire the famous yew trees of the Cotswolds. They could easily be mistaken for ordinary, enchanted tourists.
Then they stop at Dr. Jenner’s House, a museum dedicated to educating the public about the life of Gloucestershire scientist Edward Jenner. In 1796, Jenner inoculated an eight-year-old boy against smallpox with a sample collected from a cowpox sore on the hand of a milkmaid. In the following years, Jenner helped popularize vaccination, essentially founding the field of immunology.
On this day in 2023, the museum is closed. But when the scientists find a staff member and explain why the visit is so important to them, they receive a private tour of the house and grounds. That’s because the tourists, Arlene Sharpe, MD ’82 PhD ’81, and Gordon Freeman, PhD ’79, are not just scientists but immunologists. Indeed, if Edward Jenner was the “father of immunology,” as he is often called, Sharpe and Freeman are among the heirs to his legacy.
For Sharpe and Freeman, the museum is one stop in a lifetime of scientific exploration. Sharpe, the chair of the Harvard Medical School Department of Immunology and Kolokotrones University Professor, and Freeman, an HMS professor of medicine at Dana-Farber Cancer Institute, have been married for forty-seven years and professional collaborators for almost as long. All the while, they’ve brought complementary skills and insights to the study of the immune system: Freeman through his expertise in molecular biology, and Sharpe through her use of knockout technologies, genetic engineering techniques used to inactivate or remove specific genes in an organism. Their research on cell communication systems involved in immune responses has led to a fusion of their two approaches that directly contributed to the development of immune checkpoint inhibitors — a form of immunotherapy that stimulates the immune system’s natural defenses to ward off cancer.
Their work has contributed to the development of an entirely new class of cancer therapies and to a deeper understanding of how the immune system works, opening new portals to discovery. Together, their careers represent a journey through a highly productive period in the history of American biomedical research.
From the Heartland to Harvard
As a child growing up in northwest Indiana in the 1950s and ’60s, Sharpe conducted hydroponics experiments in her living room. Though she remembers succeeding only in growing mold, her parents supported her curiosity.
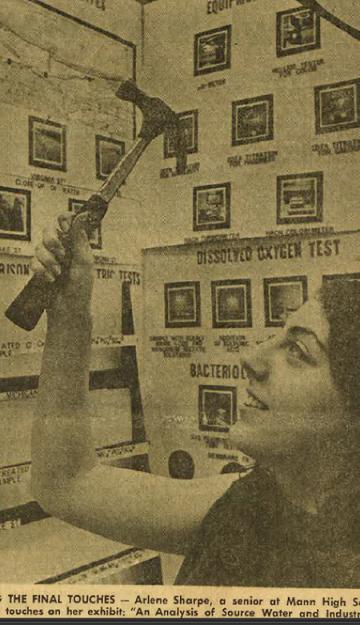
When Sharpe was fifteen, her mother died of cancer, a loss that left a deep impression. That experience motivated her to pursue a career in science. In high school, she took part in the Westinghouse Science Talent Search, a prestigious nationwide competition, working on a project analyzing samples at a local water company. When she graduated, Sharpe headed east to Harvard to take the next step toward becoming a scientist.
As a high-schooler in a suburb of Fort Worth, Texas, Freeman also took part in the Westinghouse competition, becoming a finalist two years before Sharpe entered it. In his high school, a science teacher had built a lab where students could do research, funded by a National Science Foundation (NSF) grant to encourage scientific education. “It was one of these old ramshackle wooden buildings behind the high school, but it had a complete biology lab with spectrophotometers, a dark room, and autoclaves,” Freeman says. The same teacher convinced him to apply to a summer research program at the University of Texas funded by the NSF. Freeman was accepted, helping prepare him to pursue science when he arrived at Harvard as an undergraduate.
It wasn’t just a coincidence that Sharpe and Freeman both had opportunities to explore their scientific interests at a young age. This was the post-Sputnik era, when the United States was obsessed with keeping up with the Soviet Union — not just militarily but scientifically and technologically. Sharpe and Freeman were among the many children and teenagers who benefited from a massive influx of funding for science education. Over just one year, from 1958 to 1959, NSF funding more than doubled, increasing from $52 million to $138 million. By 1975, NSF funding surpassed $800 million.
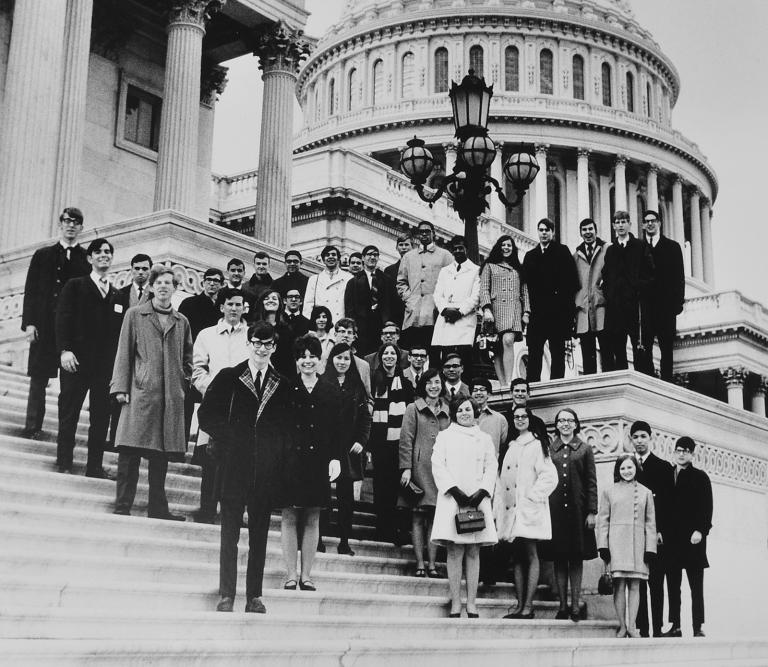
Sharpe and Freeman met when they took the same German class at Harvard. “At that point in my life, I was a very, very late-night person,” Freeman says. “It would be two hours of sleep and then German class. Sometimes I didn’t make it.”
“Gordon would come over and ask what the assignments were,” Sharpe says. “After a while, I realized he was interested in more than just the assignments.”
Freeman says Sharpe’s “animation and curiosity” piqued his interest. And the University as a whole — not just Freeman — embraced her intellectual eagerness. “I was the Indiana girl,” Sharpe says. “Coming to Harvard and Radcliffe was life-changing because I met other women who had similar aspirations.”
After graduation, Sharpe planned to attend medical school. She contemplated attending Yale School of Medicine instead of continuing at Harvard. Wanting to make sure they stayed together, Freeman proposed, and they remained in Boston.
The family business
After earning their bachelor’s degrees, Sharpe and Freeman pursued their separate but related scientific interests — Sharpe as an MD-PhD student and Freeman as a graduate student in the HMS Department of Microbiology and Molecular Genetics. For her graduate work in the lab of Bernard Fields, Sharpe studied how viruses cause diseases in their target hosts, with a focus on reoviruses, a type of RNA virus that is a versatile model for studying pathogenesis. This experience later inspired Sharpe to pursue pathology as a resident at Brigham and Women’s Hospital.
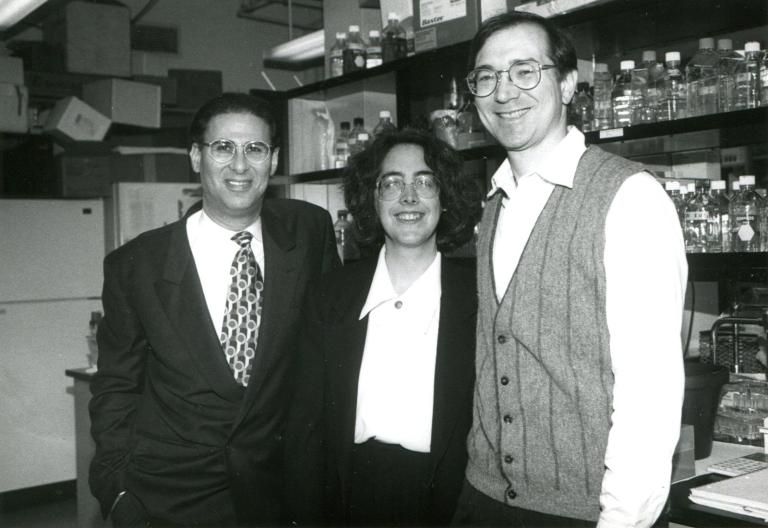
As a postdoctoral fellow, Sharpe worked with Rudolph Jaenisch at the Whitehead Institute during the early days of knockout approaches — which can be thought of as precursors to CRISPR-Cas9 gene editing. At that time, “every step of making a knockout was a Nature paper,” Sharpe says.
Freeman went in a different direction. During his postdoctoral fellowship at Dana-Farber, he explored gene cloning, a new technology at the time, working alongside Harvey Cantor (now the Baruj Benacerraf Professor of Immunology at Dana-Farber) and later Lee Nadler, MD ’73 (now the HMS Virginia and D.K. Ludwig Professor of Medicine at Dana-Farber). “Molecular biology really blossomed” in concert with the advent of gene cloning, Freeman says. It allowed scientists to identify and manipulate specific immune-related genes, whereas in the past, immunology had relied on observational studies and broader analyses of immune responses.
One gene Freeman cloned in 1989 codes for the B7 protein (also known as CD80), which has a critical role in the immune system. When a pathogen infects the body, there may be just fifty to one hundred T cells capable of recognizing that specific pathogen. B7 contributes to expanding that T cell population from fifty to perhaps fifty million, creating “an effective fighting force,” as Freeman describes it. B7 has the same role in expanding cancer-fighting cells.
Freeman and his colleagues at Dana-Farber tried inserting B7 into cancer cells to see if it would stimulate an immune response against the cancer. This worked in mice but not in human trials. Rather than abandon B7, he turned to Sharpe’s expertise in knockout technology. She developed a mouse model that lacked B7, a fortuitous step that led to the discovery of genes related to B7, including B7-2 (also known as CD86). Their 1993 paper in Science describing this work was their first joint publication and launched what Freeman likes to call “the family business.” They then extended their studies to the functions of additional members of the B7 family.
Sharpe and Freeman embarked on another type of family business around the same time: parenthood. Their children, Sam and Suzanne, were born as Sharpe and Freeman were ramping up their early discovery work. Sharpe joined the HMS faculty as an assistant professor of pathology in 1991, and Freeman started his lab as an HMS assistant professor of medicine at Dana-Farber in 1994.
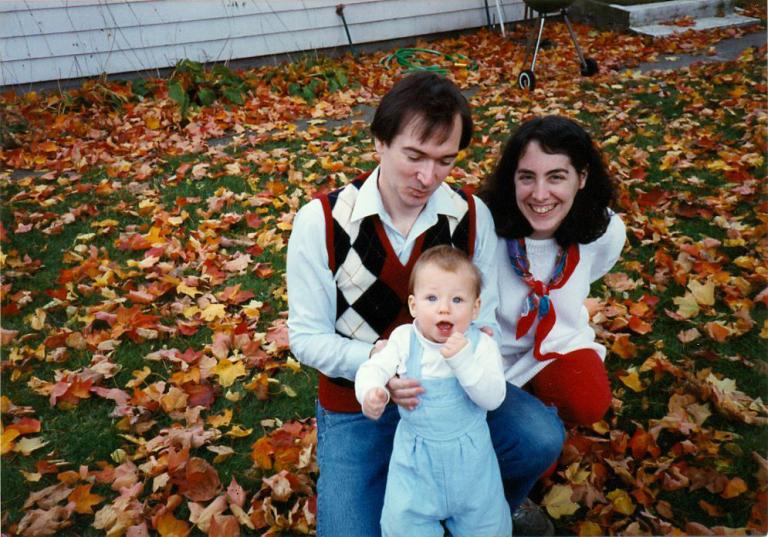
Balancing the demands of childcare and the complexities of biomedical research proved difficult. “I would be going back and forth to the lab and home, working on experiments, trying to write papers,” Sharpe says. “It was an exciting but challenging time.”
One of Sharpe’s mentors, pathologist Ramzi Cotran — with whom she had worked during residency — wasn’t shy about asking her what she needed. “He said, ‘I can’t pretend to know what you’re going to need to navigate this, but we have other women faculty who have done this. Let me introduce you to them,’" Sharpe says.
Cotran’s introductions were crucial. It wasn’t easy for women faculty to meet other women faculty. Elizabeth Petri Henske, MD ’85, now an HMS professor of medicine at Brigham and Women’s, remembers being a junior faculty member in the 1990s and seeing Sharpe in the halls of the hospital.
“I could go a month and never even pass in the hallway a woman senior to me,” Henske says. “There were just so few of them.”
At the time Sharpe and Freeman were exploring B7, cancer immunology “hadn’t produced cures,” Freeman says, “just promise.” Indeed, over the course of the twentieth century, sentiment regarding even the possibility of co-opting the immune system to fight cancer vacillated between optimism and skepticism.
About a century before Sharpe and Freeman were establishing their labs, New York surgeon William Coley reported remarkable success in treating cancer by injecting Streptococcus bacteria (“Coley’s Toxins”) into more than a thousand patients over the course of several decades. But attempts to replicate his findings failed, and interest waned as more effective treatments such as chemotherapy and radiation emerged.
The 1970s saw a surge of interest nationally in cancer research. President Nixon announced the War on Cancer in 1971, and the National Cancer Institute (NCI) budget more than doubled from 1970 to 1972. But it would be quite some time before the scientific community reawakened to the potential of cancer immunotherapy. As recently as the early 2000s, the NCI and biopharmaceutical companies were largely focused on targeted kinase inhibitors (drugs that block enzymes called kinases in cancer cells, impeding their growth).
But the fact remained: Existing cancer treatments — chemotherapy, radiation, surgery, and even kinase inhibitors — were neither sufficient nor practical for many patients.
Immunologist and Nobel Laureate Baruj Benacerraf, Dana-Farber’s president from 1980 to 1992, attracted top scientific talent and raised funding through philanthropy, leading the institution through a period of significant growth. Under his leadership, Dana-Farber became an “immunologically focused place,” Freeman says. “It was good about keeping a wide range of possibilities in play.”
That commitment — plus consistent funding for basic science research from the National Institute of Allergy and Infectious Diseases and, later, the NCI — put Sharpe and Freeman in the right place at the right time.
Lock and key
A breakthrough in a new approach to harnessing the immune system against cancer came in 1996, when James Allison, a scientist who was then at the University of California, Berkeley, demonstrated that antibodies against the CTLA-4 protein could eliminate tumors in mice. Around the same time, Sharpe developed a mouse model that lacked CTLA-4 and revealed that CTLA-4 acts as a brake on T cells. These findings focused attention on other similar targets, including one called PD-1, which had been discovered by Japanese scientist Tasuku Honjo in 1992 and would soon prove to be pivotal.
In the late 1990s, Sharpe and Freeman became intrigued by a member of the B7 family Freeman identified called PD-L1 (originally called B7-H1). In collaboration with others, Freeman’s lab discovered that, unlike other well-studied members of the B7 family, PD-L1 suppressed — rather than stimulated — the immune system by interacting with PD-1.
“It was an electric atmosphere. You knew the revolution had arrived.”
The critical finding, published in 2000 in the Journal of Experimental Medicine, was this: When PD-L1 binds to PD-1 receptors on T cells, it activates an inhibitory pathway that puts the brakes on T-cell activity. This is why immunologists refer to PD-1 as an immune checkpoint protein. Freeman and Sharpe showed that when PD-1 is engaged by PD-L1 (or its sibling, PD-L2), PD-1 curbs T cells’ supercharged powers so they don’t act as aggressors toward the body’s own tissues and organs.
“This was the lock and key to turn off the immune system,” Freeman says.
He and Sharpe demonstrated in subsequent years — along with Honjo and others — that blocking the PD-1/PD-L1 interaction could trigger an antitumor response. Further research revealed the inner workings of this lock-and-key system: By cloaking themselves in PD-L1, cancer cells render T cells inert and helpless, ensuring the cancer cells’ survival. To make matters worse, a 2002 discovery showed that when T cells recognize a tumor, they produce a protein called interferon-gamma, which raises PD-L1 levels — thereby inadvertently pressing on the brakes even harder.
But disrupting the interaction between PD-1 and PD-L1 with antibodies could disable cancer cells’ sneaky tactics and restore the power of T cells to destroy them. Hence the fundamental principle of checkpoint blockade immunotherapy: By blocking the inhibitory pathways of PD-1/PD-L1, the brakes on T-cell activity could be released, and a full-fledged attack on cancer could commence.
Dana-Farber licensed patents on Freeman’s and Sharpe’s discoveries nonexclusively, meaning multiple companies could try their hand at developing PD-1/PD-L1 antibodies.
At the 2014 meeting of the American Society of Clinical Oncology in Chicago, Sharpe gave the opening keynote address about PD-1 biology, and then representatives from pharmaceutical companies Bristol Myers Squibb, Genentech, and Merck reported clinical trial results for their PD-1/PD-L1 antibodies — which have since been approved by the U.S. Food and Drug Administration as the drugs nivolumab, atezolizumab, and pembrolizumab, respectively. Whereas just one year before, checkpoint blockade had only been successful in advanced melanoma (through the drug ipilimumab, which inhibits CTLA-4 and was approved by the FDA in 2011), the new results indicated a watershed moment, with success in non-small cell lung cancer, renal cell carcinoma, and urothelial bladder cancer.
“People were literally crying and hugging each other,” Sharpe says.
Freeman agrees. “It was an electric atmosphere. You knew the revolution had arrived.”
Immune checkpoint inhibitors are now approved for more than twenty-five types of cancer. For melanoma patients, checkpoint blockade has radically changed outcomes. Before these therapies, few people survived metastatic melanoma for more than two years. But in a recent clinical trial, about half of patients with advanced melanoma who were treated with a combination of checkpoint inhibitors were still alive after ten years.
The drugs that treat these patients are among the best-selling in the world, and they are built on the premise that a patient’s own immune system is the strongest medicine.
It’s a beautiful message, and yet, cancer immunotherapies aren’t foolproof; about 14 percent of patients treated with PD-1 or PD-L1 inhibitors experience a serious adverse event. Nevertheless, the advantages of immunotherapy over chemotherapy, for example, are stark: Side effects are, in general, less severe with immune checkpoint inhibitors than with chemotherapy and, overall, the treatment is more durable and has a higher success rate.
“Chemotherapy is one of the great dreads of the latter stage of American life,” Freeman says. “Immunotherapy is kinder and gentler.”
The ultimate journey
To bring the benefits of immunotherapy to more people, Sharpe and Freeman are studying so-called combination approaches. That is, they’re looking into why certain combinations of immunotherapy and other therapeutic options, as well as the blockade of multiple inhibitory pathways at once, work well in some patients and not others. They’re also analyzing the complexities of the tumor microenvironment, including the effects of the microbiome on patient responses to immunotherapy. Finally, they’re teaming up with colleagues to understand how their immunological insights can inform autoimmunity, aging, and tissue inflammation. These are all topics of relevance to the new Gene Lay Institute of Immunology and Inflammation, a collaboration among Brigham and Women’s, Massachusetts General Hospital, and HMS that has Sharpe as a vice director.
Meanwhile, they’ve also gained tremendous respect from their peers. “Arlene and Gordon are incredibly insightful, energetic, and enthusiastic scientists, collaborators, and educators,” says Dennis Kasper, the William Ellery Channing Professor of Medicine and a professor of immunology at HMS. “They each bring a unique skill set to their work, and the synergy of their efforts results in important contributions impacting all of medicine and science.”
“Their technical expertise is very complementary,” says Rafi Ahmed, PhD ’81, who went to graduate school with Sharpe and is now a professor in the department of microbiology and immunology at Emory University. “And they’re both deep thinkers and outstanding scientists.”
Just as Sharpe and Freeman’s lock-and-key collaborations have spurred an extraordinary wave of new research, they know the journey won’t end when they someday hang up their lab coats. Nurturing the next generation is critical — not just because mentorship is good for trainees’ career growth, but because it’s the way of science.
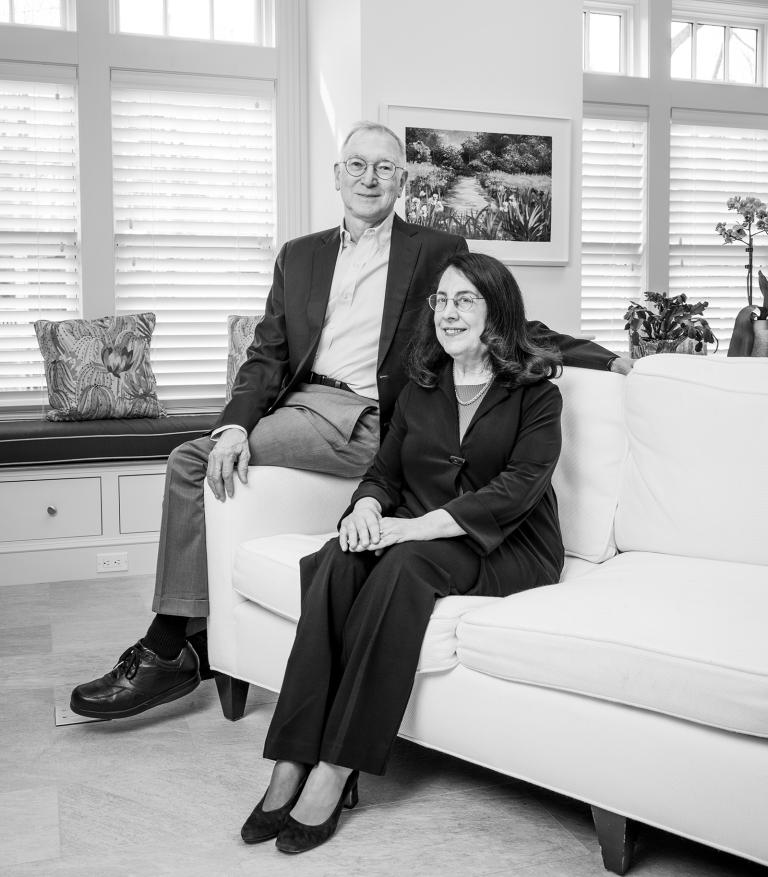
“Our students and postdocs can be human bridges to create new fields,” says Sharpe, who has been a staunch advocate for the value of mentorship.
“Arlene was always the first person I would turn to when facing tough moments in my career,” says Kristen Pauken, an assistant professor in the department of immunology at the University of Texas MD Anderson Cancer Center and a former postdoc in Sharpe’s lab. “She’s a one-in-a-million kind of mentor.”
Henske, who studies tuberous sclerosis complex, a rare genetic disorder that causes tumors to form in various organs, feels indebted to Sharpe and Freeman for their basic science discoveries.
“They weren’t trying to treat a disease,” Henske says. “They and others were trying to understand: How is the activity of T cells regulated? Now this work on immune checkpoints is benefitting many people who are living with cancer. And it wouldn’t have happened without people like Arlene and Gordon revealing the basic mechanisms.”
For Sharpe and Freeman, curiosity and a love of discovery are evident in all aspects of their life together. Besides the Cotswolds, recent trips include the Galapagos — Darwin’s famous scientific playground — and the Lyngen Alps in Norway to see the aurora borealis.
They both know, however, that science itself is the ultimate adventure.
“Funding curiosity-driven science is so important,” Sharpe says. “It takes you down avenues you could never have imagined.”
Allison Eck is the executive communications manager in the HMS Office of Communications and External Relations.